Volume 29, No.3 Pages 269 - 276
3. SPring-8/SACLA通信/SPring-8/SACLA COMMUNICATIONS
利用系活動報告
Activity Report of the Precision Spectroscopy Division 2024
(公財)高輝度光科学研究センター 放射光利用研究基盤センター 精密分光推進室 Precision Spectroscopy Division, Center for Synchrotron Radiation Research, JASRI

1. Introduction
The Precision Spectroscopy Division (PSD), http://www.jasri.jp/organization/organization-research-section/precision.html, focuses on meV-resolved inelastic X-ray scattering (IXS) and nuclear resonant scattering (NRS). These two areas of research are somewhat outside the historical mainstream of X-ray techniques, but they have flourished at modern synchrotron radiation sources. IXS investigates atomic dynamics (phonons in crystals, excitations in disordered materials) via measurement of the dynamic structure factor on ~1-100 meV energy scales at ~1-100 nm-1 momentum transfers (see [1][1] A. Q. R. Baron: in Synch. Light Srcs. & FELS, edited by E. J. Jaeschke, S. Khan, J. R. Schneider, and J. B. Hastings (Springer International Publishing, Cham, 2016), pp. 1643-1757.
https://doi.org/10.1007/978-3-319-14394-1
See also https://doi.org/10.48550/arXiv.1504.01098 and references therein). While in principle this overlaps some ranges accessible by inelastic neutron scattering (INS), IXS offers important advantages, including access to samples that are several orders of magnitude smaller (from ~gram scales for INS to sub-microgram for IXS), dramatically improved data for disordered materials, as well as generally cleaner data than INS. NRS offers a variety of techniques (see [2, 3][2] E. Gerdau and H. de Waard: Hyperfine Interact. 123-125 (1999-2000).
[3] M. Seto: J. Phys. Soc. Japan 82 (2013) 021016.
https://doi.org/10.7566/JPSJ.82.021016 and references therein) that probe atomic, magnetic and electronic structure and dynamics on energy scales from neV to meV. While the NRS methods do have some overlap with those of classical Mössbauer spectroscopy (MS), NRS greatly extends the range of available methods, including access to phonon densities of states and to neV-scale atomic dynamics, and to resonances that are not easily accessible with radioactive sources.
The methods employed by the PSD, IXS and NRS, provide detailed and important information that can be difficult or impossible to obtain using other methods. These are therefore actively pursued at SPring-8 and, indeed, at most of the large synchrotron radiation facilities including ESRF, APS, PETRA (NRS only) and NSLS-II (IXS only). In this context SPring-8 has world-leading IXS spectrometers[1][1] A. Q. R. Baron: in Synch. Light Srcs. & FELS, edited by E. J. Jaeschke, S. Khan, J. R. Schneider, and J. B. Hastings (Springer International Publishing, Cham, 2016), pp. 1643-1757.
https://doi.org/10.1007/978-3-319-14394-1
See also https://doi.org/10.48550/arXiv.1504.01098. Meanwhile the NRS program has benefitted from a long tradition of NRS work in Japan[4][4] S. Kikuta, Y. Yoda, K. Izumi, K. Hirano, N. Horiguchi, T. Ishikawa, X. W. Zhang, H. Sugiyama, M. Ando, M. Seto, C. K. Suzuki, and S. Nasu: in X-Ray Reson. Scatt., edited by G. Materlik, C. J. Sparks, and K. Fischer (Elsevier, Amsterdam, 1992), pp. 635-646., including a strong focus on instrumentation development[5][5] T. Ishikawa, Y. Yoda, K. Izumi, C. K. Suzuki, X. W. Zhang, M. Ando, and S. Kikuta: Rev. Sci. Instrum. 63 (1992) 1015-1018.
https://doi.org/10.1063/1.1143188.
The PSD division goals remain, in order of priority:
1a. Maintain and improve the complex instrumentation and methods available in the division.
1b. Aid external scientists (users) to productively use the division resources to do new science.
2. Carry out and publish research, preferably using PSD instrumentation, but also extending to other instruments at SPring-8, and, perhaps, in rare cases, outside SPring-8.
3. Promote division activities and attract new users.
2. Nuclear Resonant Scattering (NRS)
The nuclear resonant scattering program includes many techniques and a rather large suite of optics and detectors. Energy scales from neV to μeV to meV can be probed, depending on the method, and X-ray energies from 14 to > 70 keV are used. The common feature is the use of long-lived (> ns lifetime) nuclear or "Mössbauer" resonances. The measurements often require timing mode / few-bunch operation of the storage ring, either to permit direct time domain measurements of the impulse response of a system, or, more often, to allow separation between nuclear scattered events and (faster) electronic scattering. However, an increasing number of measurements (see sections 2.2 and 2.4) can be done in any bunch mode. The broad range of techniques and large energy range of the resonances as well as the combination of sophisticated optics and detectors makes NRS a challenging field for user support.
2.1 Successful Program Upgrade
The NRS program moved its home base from BL09XU to BL35XU in 2021. Many of the planned changes were detailed in the last PSD activity report[6][6] 依田芳卓、内山裕士、石川大介、永澤延元、バロンアルフレッド: SPring-8/SACLA利用者情報 26 (2020) 329-334.
https://user.spring8.or.jp/sp8info/?p=39523. Here we note the changes have generally gone according to plan and have led to improved capability. In particular, the short period ID installed at BL35XU for IXS, yielded an improvement of between 2 and 3 times the flux for all setups for NRS (see table 1) compared to BL09XU. (The energy gap between ~28 and 43 keV has not been a problem). However, despite the fact that essentially all experiments are flux limited and the flux doubled or tripled, there was little or no change in the number of shifts requested by users in their proposals. This may reflect the small and relatively stable user community as well as the flux limited nature of the work.
Table 1 Flux and flux increase using the 20 mm period ID at BL35XU.
Nuclei | Energy [keV] (Bandwidth) |
Flux [GHz] |
Factor [BL35XU/BL09XU] |
57Fe | 14.4 (6 meV) | 160 | 2.1 |
14.4 (3.5 meV) | 53 | 1.9 | |
14.4 (2.5 meV) | 33 | 2.4 | |
14.4 (0.8 meV) | 5.6 | 2.1 | |
151Eu | 21.5 (1.2 meV) | 15 | 3.2 |
119Sn | 23.9 (1.5 meV) | 3.8 | 3.4 |
61Ni | 67.4 (~0.35 eV) | 74 | 2.3 |
Switching between NRS setups has become easier due to the more convenient optical layout. Also, the new toroidal mirror, similar to one installed at BL43LXU, provides a small (50 × 28 μ2, FWHM) beam at 14.4 keV and also has been used to focus at higher energy (e.g. 76 keV) though with a larger focal spot size. The measured focus (after the mirror was repolished, as the initial polishing was somehow very poor) is shown in figure 1. The one-sided tail in the vertical is mostly the effect of the high-resolution monochromator.
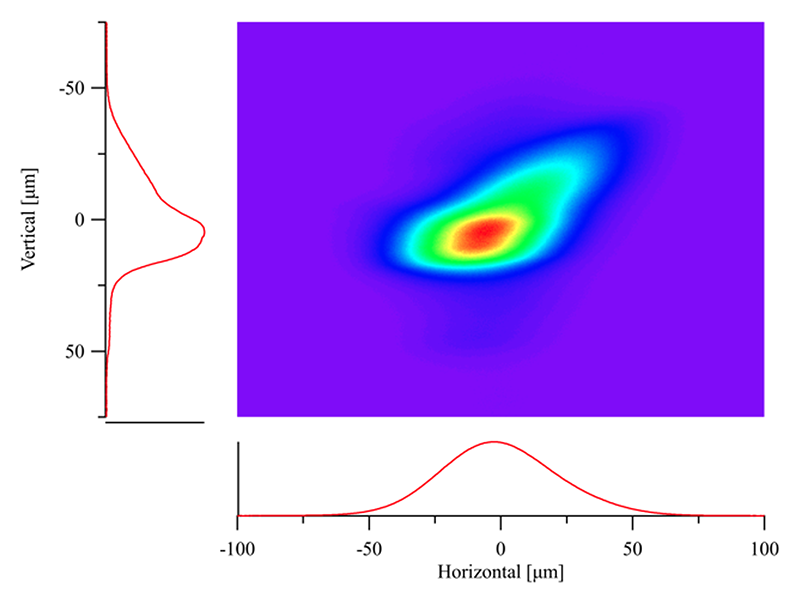
Figure 1 Focal spot at 14.4 keV from the bent cylindrical mirror c.
Other spectrometers for time domain and energy domain Mössbauer spectroscopy, nuclear inelastic scattering, and time domain interferometry were (re-)installed successfully in 2021A and subsequent periods, as originally planned, in part with greatly appreciated help from JASRI visiting researchers. Further, though not officially part of the upgrade, a new closed cycle cryostat system for NRVS experiments was installed at BL19LXU. This is much more convenient and inexpensive to run than the previous liquid He system.
2.2 Development of a SMS system
Most NRS methods require timing mode operation of the storage ring. However, if a pure nuclear reflection in a highly perfect crystal is available, it is possible to use what has been called a "Synchrotron Mössbauer Source" (SMS) setup[7][7] G. V. Smirnov, U. van Bürck, A. I. Chumakov, A. Q. R. Baron, and R. Rüffer: Phys. Rev. B 55 (1997) 5811.
https://doi.org/10.1103/PhysRevB.55.5811. That setup may be used independent of the storage ring filling pattern/timing mode. It uses the pure nuclear reflection to select only the resonant radiation, and then allows conventional ("Mössbauer") velocity scans for hyperfine spectroscopy. Hyperfine spectroscopy allows one to probe local magnetic and electronic structure, and even can provide motional information – and is a versatile probe for physics and materials science. The SMS setup will allows hyperfine spectroscopy to be done with a highly collimated beam, and, eventually, sub-micron focusing.
Over the last years, the PSD, (especially N. NAGASAWA[8][8] N. Nagasawa, Y. Yoda, and A. Q. R. Baron: Work in Progress.) has been involved in making an SMS system for use at BL35XU. This will use a slightly different geometry than is usual, employing Bragg scattering[9][9] T. Mitsui, M. Seto, R. Masuda, K. Kiriyama, and Y. Kobayashi: Jpn. J. Appl. Phys. 46 (2007) L703.
http://dx.doi.org/10.1143/JJAP.46.L703, and in particular, backscattering from in a sapphire crystal to avoid having to move the small and relatively fragile borate crystal. Recent work has included checks of a precise air-bearing stage for moving the sapphire crystal without much deviation (small pitch and yaw) and most recently installation of a furnace to control the sapphire crystal temperature, which has recently achieved < 1 mK rms temperature stability. The setup is shown in figure 2. First tests with X-rays are expected in 2024.
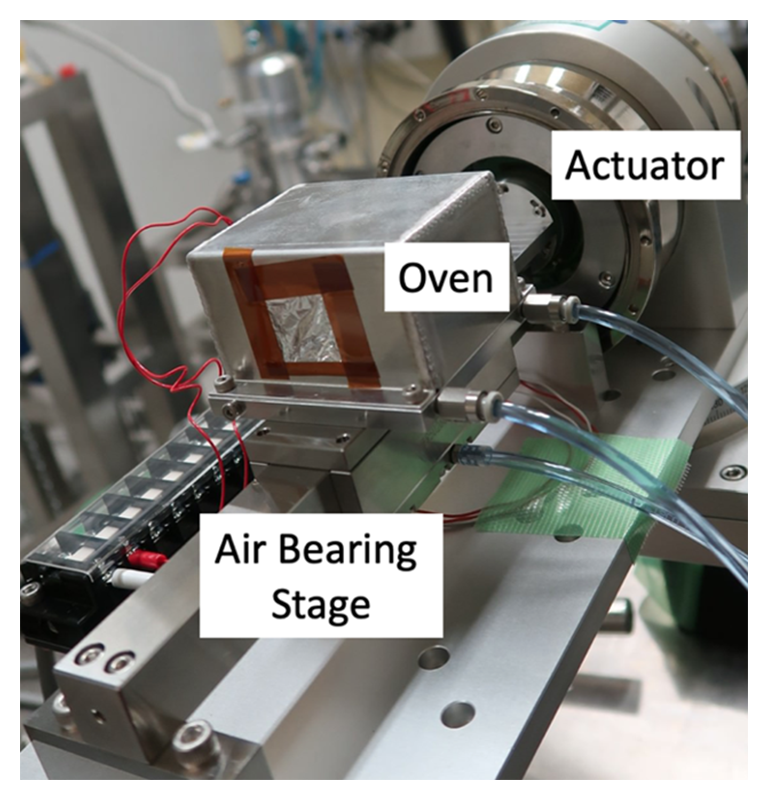
Figure 2 Part of the SMS setup showing the oven for a sapphire backscattering crystal on an air bearing stage with a "Mössbauer" velocity drive actuator. The tilt in the picture is purely an optical effect of the photograph and the motion is in the horizontal plane.
2.3 Energy Domain Mössbauer Spectroscopy
The SPring-8 NRS program has a unique focus on a method that allows spectroscopy of more exotic nuclei. This technique[10][10] M. Seto, R. Masuda, S. Higashitaniguchi, S. Kitao, Y. Kobayashi, C. Inaba, T. Mitsui, and Y. Yoda: Phys. Rev. Lett. 102 (2009) 217602.
http://dx.doi.org/10.1103/PhysRevLett.102.217602, which we call "Energy Domain Mössbauer Spectroscopy" (EDMS) facilitates hyperfine spectroscopy measurements on isotopes that are difficult to access otherwise. It has been applied to resonances including 61Ni, 99Ru, 149Sm, 151Eu, 161Dy, 174Yb, 193Ir. In general, it provides information that is similar to more conventional hyperfine spectroscopy. However, the method does require timing mode operation and also tends to have long (often > 8 hours per spectrum) collection times. At SPring-8 there has been an interest in measurement of both time and velocity histograms to, eventually, improve the reliability of the analysis. Building on extensive earlier work and the purchase of cutting edge electronics, recent work (mostly by N. NAGASAWA) has focused on simplifying the data collection.
2.4 Energy Domain Quasi-Elastic Scattering
There has been an ongoing collaboration between Tohoku University, RIKEN and PSD staff, among others, to make an energy domain spectrometer with sub-μeV resolution. This is essentially a synchrotron version of an older of technique called "Rayleigh Scattering of Mössbauer Radiation" [11][11] Champeney, D. C.: Rep. Prog. Phys. 42 (1979) 1017-1054.
https://doi.org/10.1088/0034-4885/42/6/002 but leverages both the more intense beams of the SR source and the advantages of the CITIUS area detector to allow, potentially, powerful measurements over a large range of momentum transfers (see figure 3 but note that one expects the indicated range of momentum transfers for the MLS setup is conservative), and in any bunch mode.
First measurements were recently published[12][12] Saito M. et al.: Phys. Rev. Lett. 132 (2018) 256901.
https://doi.org/10.48550/arXiv.1807.03620 and the setup should provide a much-needed window intoinvestigations of glass forming liquids and polymers.
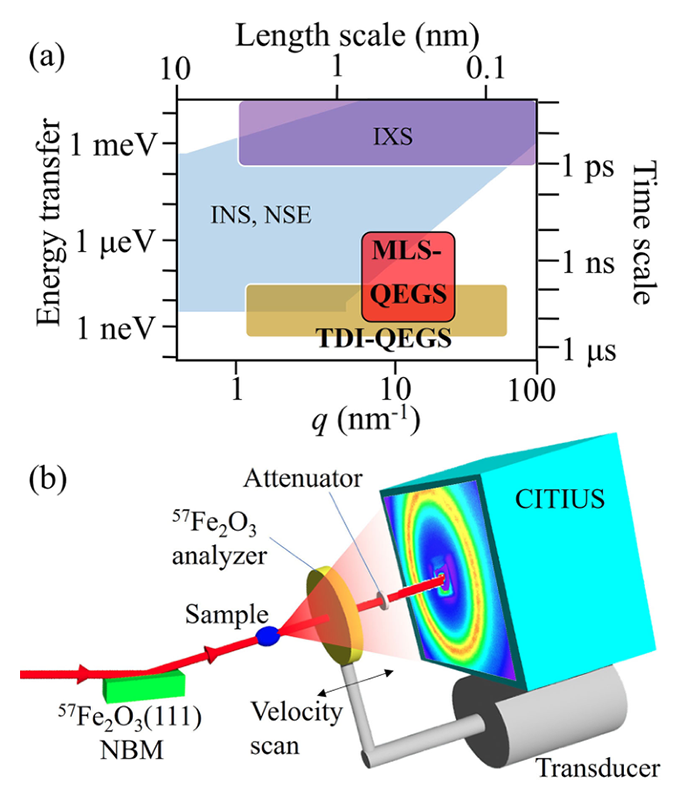
Figure 3 Setup for energy domain quasi-elastic scattering (lower) and expected range of application (box labeled MLS-QEGS) (upper) from [12][12] Saito M. et al.: Phys. Rev. Lett. 132 (2018) 256901.
https://doi.org/10.48550/arXiv.1807.03620.
3. Inelastic X-Ray Scattering (IXS)
IXS is used to investigate atomic dynamics in materials. There are several standard setups offered at BL35XU[13][13] A. Q. R. Baron, Y. Tanaka, S. Goto, K. Takeshita, T. Matsushita, and T. Ishikawa: J. Phys. Chem. Solids 61 (2000) 461.
https://doi.org/10.1016/S0022-3697(99)00337-6 including a > ~1.4 meV resolution setup using the Si(11 11 11) reflection at 21.7 keV, and ~3 meV resolution setup using the Si(9 9 9) reflection at 17.8 keV. These can either be used with standard focusing with an ~80 micron beam size or with compound focusing where KB mirrors are used to reduce the size to < 20 microns[14][14] D. Ishikawa, H. Uchiyama, S. Tsutsui, H. Fukui, and A. Q. R. Baron: SPIE 8848 (2013) 88480F.
https://doi.org/10.1117/12.2023795. The latter is used with diamond anvil cells (DACs) to investigate materials under extreme pressure. The experimental opportunities for IXS at BL43LXU[15][15] A. Q. R. Baron: SPring-8 Infomation 15 (2010) 14.
https://user.spring8.or.jp/sp8info/?p=3138 are similar to those at BL35XU, but the flux at BL43LXU is higher, with more analyzers and a smaller beam size (see also [1][1] A. Q. R. Baron: in Synch. Light Srcs. & FELS, edited by E. J. Jaeschke, S. Khan, J. R. Schneider, and J. B. Hastings (Springer International Publishing, Cham, 2016), pp. 1643-1757.
https://doi.org/10.1007/978-3-319-14394-1
See also https://doi.org/10.48550/arXiv.1504.01098 and section 3.4, below).
The applicable scientific fields for IXS are wide, as phonons and atomic dynamics are related to many material properties. Relevant fields include atomic dynamics in liquids and glasses, electron-phonon coupling in correlated materials, including superconductors, sound velocity and elastic constants in extreme conditions (pressures in excess of 300 GPa and temperatures above 3000 K have been achieved in IXS), which are often correlated with seismological work, phonon lifetimes in thermoelectric materials, magneto-elastic coupling, etc. In the following, we focus mostly on instrumentation results related to improvements in capability.
3.1 Thin Films
There has been an ongoing project at BL35XU to investigate thin film samples. This allows access to structured/layered materials as are often interesting to control thermal transport, and also to interface-stabilized materials that are simply not available in bulk. However, investigating thin films samples requires some specialized setups, as one usually must do experiments in a grazing incidence geometry to increase the X-ray path length in the film. This means there must be precise control over the sample positions, grazing angles, and also good calculations as the grazing angle is comparable with the angular deviations of the incident beam out of the horizontal spectrometer scattering plane caused by the focusing optics (the bent cylindrical mirror, or, the KB optics). The last means that the errors of the conventional 4-cricle geometry code are not negligible: calculations need to be done in a 6-circle geometry.
Building on the work in the materials Dynamics Group[16][16] W. Zhao and A. Q. R. Baron: (2020) Unpublished., H. FUKUI and T. MANJO have created software that allows general HKL scans to be done using SPEC. The underlying Python 3 based six-circle code[16][16] W. Zhao and A. Q. R. Baron: (2020) Unpublished. numerically solves the diffraction conditions for the 6-circle angles 2Θ, θ, φ, χ, γ, μ, and the surface angles, α and β, with the option to hold a subset of them (or ω) constant. It also calculates the momentum transfers and momentum transfer resolution for all the analyzers in the arrays at either BL43LXU or BL35XU. The added code then allows calculation of HKL scans that can be easily loaded into the usual SPEC software. In addition, the portability of the Python 3 code allows users to investigate, before coming to SPring-8, sample orientations for measuring phonons to learn how to use the analyzer arrays effectively.
In addition to the software/control aspects, there has been work to make a furnace that is suitable for thin film work, as is shown in figure 4. Also, first tests of a LN2 refrigerator system (Anton Paar) have been carried out. A new refrigerator system is desirable as, in a grazing incidence geometry, the vibrations of the usual beamline closed cycle systems can lead, effectively, to large beam motion on the sample.
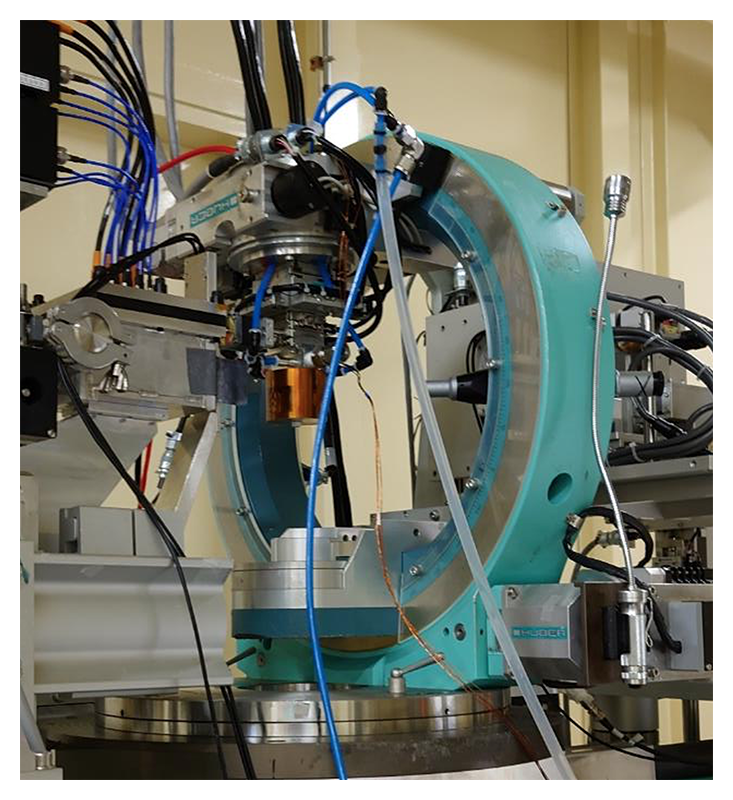
Figure 4 Furnace for making IXS measurements of thin films at grazing incidence mounted in the Eulerian Cradle at BL35XU.
3.2 Software Integration
A first goal of IXS measurements is to generate plots and data files of the scattered intensity as a function of energy transfer for each analyzer for each arm position where a measurement is made. This typically requires summing data from several scans, including conversion from the temperature to energy, normalization, and application of several calibrations/corrections that are determined at the start of the experiment. Then one must plot the spectra so the results are accessible for the full analyzer array (12 spectra at BL35XU, 28 spectra at BL43LXU).
Therefore there has been effort (primarily by T. MANJO, but also related to work by D. ISHIKAWA) to make scripts that automate the process. The new Python 3-based wrapper software uses one "list" file that contains information for all the spectra – one just adds one line for each dataset. The python code then creates the input files, transfers data around as needed, and runs the earlier processing software. This allows the data from an entire experiment to be easily processed – including a fair number of sophisticated plotting options as have been found to be useful for IXS, including overlay with the "sarf" (deconvolved resolution function, see [17][17] D. Ishikawa and A. Q. R. Baron: J. Synch. Rad. 28 (2021) 804-811.
https://doi.org/10.1107/S1600577521003234). Examples are given in figure 5.
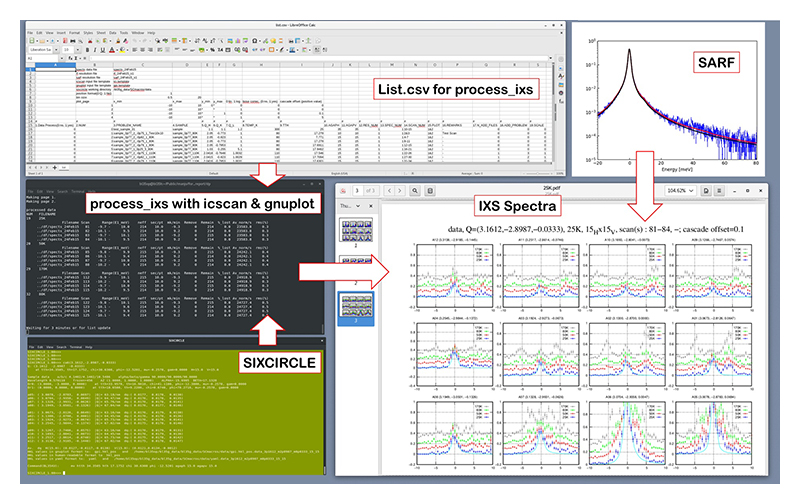
Figure 5 Integrated data processing for IXS combining several steps into a smooth an automatically updating system. A "list" with one line of input per measurement combines several processes as discussed in the text, and allows easy creation of graphics for investigating inelastic response.
3.3 New IXS Pixel Array Detectors
Area or pixel array detectors (PADs) are interesting for IXS as, in principle, they can allow improved resolution, and be relatively easy to use. However, background rates and efficiency are significant issues for these detectors, and it is difficult to match the performance of the original single element detectors (SEDs) used for IXS: 2 × 2 mm2 × 1 mm thick CZT which have background rates that are ~0.02 events/s/cm2 and are expected to be nearly 100% efficient (17-30 keV). In comparison, measurements (see [18][18] A. Q. R. Baron and D. Ishikawa: Nucl. Inst. Meth. Phys. Res. A. 1049 (2023) 168101.
https://doi.org/10.1016/j.nima.2023.168101 and references therein) with higher Z area detectors (e.g. CdTe) show background rates ~0.4 events/s/cm2, which is large enough to be a problem in low rate IXS measurements and, due to charge sharing, give efficiencies that are more like 70-80%, when one exceeds the K-edges of the detector material.
Considering these issues, and the potential improvements, a study was undertaken (mostly by A. BARON) to investigate how to reduce backgrounds and improve the practical efficiency of the detectors[18][18] A. Q. R. Baron and D. Ishikawa: Nucl. Inst. Meth. Phys. Res. A. 1049 (2023) 168101.
https://doi.org/10.1016/j.nima.2023.168101. This yielded significant improvements (see figure 6), and, finally reduction of background rates down to < 0.05/s/cm2, or with care, to < 0.005/s/cm2, and measured efficiencies > 90% without negative impact (double-counting) due to charge sharing. The SED detectors at BL43LXU have now been replaced by area detectors, leading to significant improvements in resolution for many analyzers, and easier operation[19][19] D. Ishikawa, T. Manjo, A. Q. R Baron, et al.: In Preparation.. It is expected that a similar system will be installed at BL35XU in the near future.
Incidentally, one notes that the high frame rates and low dark current (low LLD setting) that are now possible using most high-Z area detectors, means that it is easy to experimentally estimate the absolute efficiency of the detector for mid-energy (say 15-40 keV) X-rays.
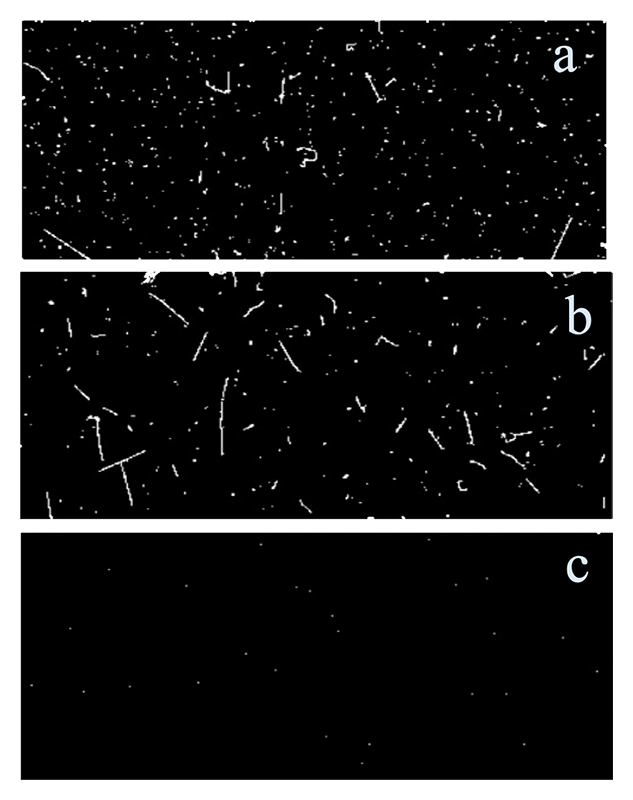
Figure 6 Background measured on the SPring-8 experimental floor in a 15 × 30 mm2 area of a 0.75 mm thick CdTe Eiger 2 pixel array detector. Each exposure was for 2000 s. (a) 0.4 events/s/cm2 without processing or shielding, (b) 0.05/s/cm2 with shielding and (c) 0.004/s/cm2 with shielding and processing. See [18][18] A. Q. R. Baron and D. Ishikawa: Nucl. Inst. Meth. Phys. Res. A. 1049 (2023) 168101.
https://doi.org/10.1016/j.nima.2023.168101.
3.4 High Pressure and a New Pressure Scale
SPring-8 continues to do meV-IXS experiments at the highest pressures world-wide, as is made possible by having the smallest, 5 μm beam size for IXS and a lot of auxiliary instrumentation[20][20] A. Q. R Baron, D. Ishikawa, H. Fukui and Y. Nakajima: AIP Conf. Proc. 2054 (2019) 20002.
https://doi.org/10.48550/arXiv.1807.03620 of BL43LXU. This has allowed results in iron to > 300 GPa, and, most recently, a new primary pressure scale[21][21] D. Ikuta, E. Ohtani, H. Fukui, T. Sakamaki, R. Heid, D. Ishikawa and A. Q. R. Baron: Sci. Adv. 9 (2023) eadh8706.
https://doi.org/10.1126/sciadv.adh8706, valid to > 200 GPa, which we show here as an example. The primary scale is important for geoscience as it is the only way to compare measurements made in lab settings with conditions expected in the interior of planets. Thus such a scale is required to understand the structure of planetary interiors, and, eventually Solar system evolution, etc.
Figure 7 shows the new scale relative to other scales, and indicates that there is a very large shift at pressures over 200 GPa – with the main previously accepted scale over estimating pressures by ~20%.
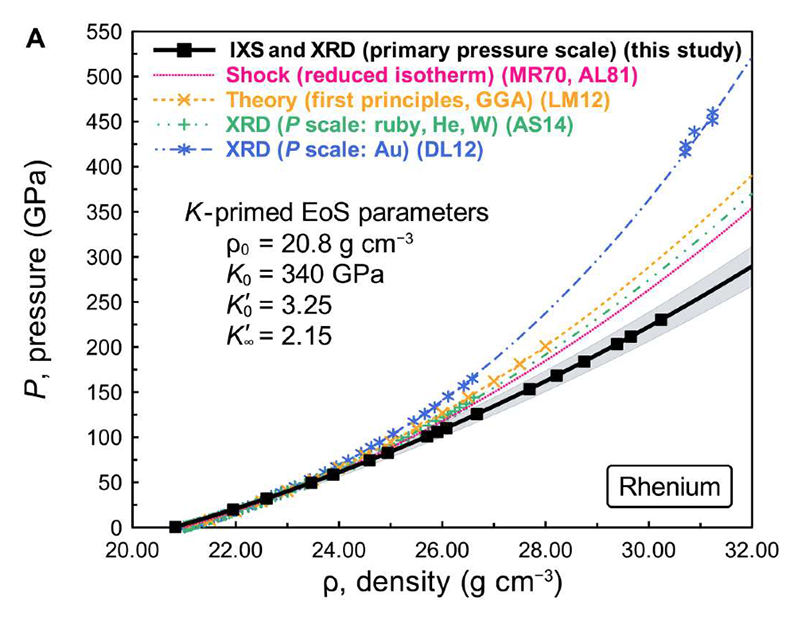
Figure 7 New primary pressure scale compared against previous scales. The grey shaded region is the new work while the other lines represent previous scales. From [21][21] D. Ikuta, E. Ohtani, H. Fukui, T. Sakamaki, R. Heid, D. Ishikawa and A. Q. R. Baron: Sci. Adv. 9 (2023) eadh8706.
https://doi.org/10.1126/sciadv.adh8706.
4. Other Facilities at SPring-8
The PSD (mostly H. FUKUI) also supports several facilities away from the beamlines. This includes (1) a focused ion beam (FIB) setup that uses Ga ions to mill out ~10 micron scale (usually single crystal) samples from larger pieces, for e.g. placing in diamond anvil cells (DACs). This setup is now rather old, but mostly continues to work. There is also (2) a "Laser Drill" that can be used to make small, 0.01-0.1 mm scale holes in materials, including Beryllium, that can then be used as gaskets for DAC preparation. Most recently, (3), a new and sophisticated XRG (figure 8) was bought that should allow nearly automatic checking of sample quality and alignment, as was of interest to several groups at SPring-8. It is now being commissioned.
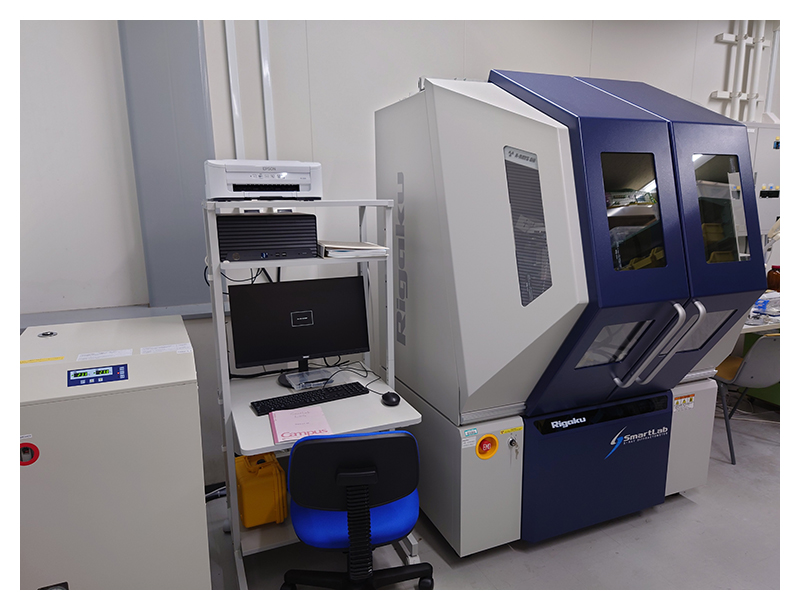
Figure 8 New highly automated and flexible X-ray generator installed in room 共通室 B3-1/b 共 07.
5. IXS2024
Finally we note that PSD is making a large contribution to the 13th International Conference on Inelastic X-Ray Scattering (IXS2024) which will be held in Himeji from 8–13 September, 2024. In addition to support from different parts of the SPring-8 community, the conference has had large support from within the PSD, with specifically, A. BARON acting as Conference Chair, with H. FUKUI, T. MANJO, N. NAGASAWA and D. ISHIKAWA all providing service as members of the local organizing committee (LOC). (see http://ixs2024.jasri.jp/)
6. Concluding Comments
The Precision Spectroscopy Division is now establishing a new equilibrium after significant changes in both beamline operations and in organization ~4 years ago. The focus of two staff (N. NAGASAWA and Y. YODA) on NRS work mostly at BL35XU, but also sometimes at BL19LXU, two staff (H. FUKUI and T. MANJO) on IXS mostly at BL35XU (and occasionally helping at BL43LXU), and two staff (D. ISHIKAWA and A. BARON) for IXS mostly at BL43LXU (but helping at BL35XU, and doing large amounts of R&D to improve IXS, and (AB) providing guidance) seems to be reasonable: this allows some forward progress in the various methods, and avoids the more severe levels of exhaustion that quickly become a problem with fewer staff. However, even this level still does not allow much time for research. It is expected that things will stabilize more over the next two to three years and more in-house research will become possible.
References
[1] A. Q. R. Baron: in Synch. Light Srcs. & FELS, edited by E. J. Jaeschke, S. Khan, J. R. Schneider, and J. B. Hastings (Springer International Publishing, Cham, 2016), pp. 1643-1757.
https://doi.org/10.1007/978-3-319-14394-1
See also https://doi.org/10.48550/arXiv.1504.01098
[2] E. Gerdau and H. de Waard: Hyperfine Interact. 123-125 (1999-2000).
[3] M. Seto: J. Phys. Soc. Japan 82 (2013) 021016.
https://doi.org/10.7566/JPSJ.82.021016
[4] S. Kikuta, Y. Yoda, K. Izumi, K. Hirano, N. Horiguchi, T. Ishikawa, X. W. Zhang, H. Sugiyama, M. Ando, M. Seto, C. K. Suzuki, and S. Nasu: in X-Ray Reson. Scatt., edited by G. Materlik, C. J. Sparks, and K. Fischer (Elsevier, Amsterdam, 1992), pp. 635-646.
[5] T. Ishikawa, Y. Yoda, K. Izumi, C. K. Suzuki, X. W. Zhang, M. Ando, and S. Kikuta: Rev. Sci. Instrum. 63 (1992) 1015-1018.
https://doi.org/10.1063/1.1143188
[6] 依田芳卓、内山裕士、石川大介、永澤延元、バロンアルフレッド: SPring-8/SACLA利用者情報 26 (2020) 329-334.
https://user.spring8.or.jp/sp8info/?p=39523
[7] G. V. Smirnov, U. van Bürck, A. I. Chumakov, A. Q. R. Baron, and R. Rüffer: Phys. Rev. B 55 (1997) 5811.
https://doi.org/10.1103/PhysRevB.55.5811
[8] N. Nagasawa, Y. Yoda, and A. Q. R. Baron: Work in Progress.
[9] T. Mitsui, M. Seto, R. Masuda, K. Kiriyama, and Y. Kobayashi: Jpn. J. Appl. Phys. 46 (2007) L703.
http://dx.doi.org/10.1143/JJAP.46.L703
[10] M. Seto, R. Masuda, S. Higashitaniguchi, S. Kitao, Y. Kobayashi, C. Inaba, T. Mitsui, and Y. Yoda: Phys. Rev. Lett. 102 (2009) 217602.
http://dx.doi.org/10.1103/PhysRevLett.102.217602
[11] Champeney, D. C.: Rep. Prog. Phys. 42 (1979) 1017-1054.
https://doi.org/10.1088/0034-4885/42/6/002
[12] Saito M. et al.: Phys. Rev. Lett. 132 (2018) 256901.
https://doi.org/10.48550/arXiv.1807.03620
[13] A. Q. R. Baron, Y. Tanaka, S. Goto, K. Takeshita, T. Matsushita, and T. Ishikawa: J. Phys. Chem. Solids 61 (2000) 461.
https://doi.org/10.1016/S0022-3697(99)00337-6
[14] D. Ishikawa, H. Uchiyama, S. Tsutsui, H. Fukui, and A. Q. R. Baron: SPIE 8848 (2013) 88480F.
https://doi.org/10.1117/12.2023795
[15] A. Q. R. Baron: SPring-8 Infomation 15 (2010) 14.
https://user.spring8.or.jp/sp8info/?p=3138
[16] W. Zhao and A. Q. R. Baron: (2020) Unpublished.
[17] D. Ishikawa and A. Q. R. Baron: J. Synch. Rad. 28 (2021) 804-811.
https://doi.org/10.1107/S1600577521003234
[18] A. Q. R. Baron and D. Ishikawa: Nucl. Inst. Meth. Phys. Res. A. 1049 (2023) 168101.
https://doi.org/10.1016/j.nima.2023.168101
[19] D. Ishikawa, T. Manjo, A. Q. R Baron, et al.: In Preparation.
[20] A. Q. R Baron, D. Ishikawa, H. Fukui and Y. Nakajima: AIP Conf. Proc. 2054 (2019) 20002.
https://doi.org/10.48550/arXiv.1807.03620
[21] D. Ikuta, E. Ohtani, H. Fukui, T. Sakamaki, R. Heid, D. Ishikawa and A. Q. R. Baron: Sci. Adv. 9 (2023) eadh8706.
https://doi.org/10.1126/sciadv.adh8706
(公財)高輝度光科学研究センター
放射光利用研究基盤センター 精密分光推進室
〒679-5198 兵庫県佐用郡佐用町光都1-1-1
TEL : 0791-58-0802 ext. 3939
e-mail : yoda@spring8.or.jp
(公財)高輝度光科学研究センター
放射光利用研究基盤センター 精密分光推進室
〒679-5148 兵庫県佐用郡佐用町光都1-1-1
TEL : 0791-58-0803 ext. 7880
e-mail : fukuih@spring8.or.jp
(公財)高輝度光科学研究センター
放射光利用研究基盤センター 精密分光推進室
〒679-5198 兵庫県佐用郡佐用町光都1-1-1
TEL : 0791-58-0802 ext. 3395
e-mail : nagasawa@spring8.or.jp
(公財)高輝度光科学研究センター
放射光利用研究基盤センター 精密分光推進室
〒679-5198 兵庫県佐用郡佐用町光都1-1-1
TEL : 0791-58-0803 ext. 3910
e-mail : manjo.taishun@spring8.or.jp
(公財)高輝度光科学研究センター
放射光利用研究基盤センター 精密分光推進室
(国)理化学研究所
放射光科学研究センター 物質ダイナミクスグループ
〒679-5148 兵庫県佐用郡佐用町光都1-1-1
TEL : 0791-58-0802 ext. 3779
e-mail : disikawa@spring8.or.jp
(国)理化学研究所
放射光科学研究センター 物質ダイナミクスグループ
(公財)高輝度光科学研究センター
放射光利用研究基盤センター 精密分光推進室
〒679-5148 兵庫県佐用郡佐用町光都1-1-1
TEL : 0791-58-0802 ext. 3883
e-mail : baron@spring8.or.jp