Volume 26, No.3 Pages 329 - 334
4. SPring-8/SACLA通信/SPring-8/SACLA COMMUNICATIONS
利用系グループ活動報告
放射光利用研究基盤センター 精密分光推進室
Activity Reports – Precision Spectroscopy Division
(公財)高輝度光科学研究センター 放射光利用研究基盤センター 精密分光推進室 Precision Spectroscopy Division, Center for Synchrotron Radiation Research, JASRI

1. Introduction
The Precision Spectroscopy Division (PSD), http://www.jasri.jp/organization/organization-research-section/precision.html, was created in May of 2020 and focuses primarily on meV-resolved inelastic x-ray scattering (IXS) and nuclear resonant scattering (NRS). These two areas of research are somewhat outside the historical mainstream of x-ray techniques, but they have flourished at modern synchrotron radiation sources. IXS investigates atomic dynamics (phonons in crystals, excitations in disordered materials) via measurement of the dynamic structure factor on ~1-100 meV energy scales at ~1-100 nm-1 momentum transfers (see [1] and references therein). While in principle this overlaps some ranges accessible by inelastic neutron scattering (INS), IXS offers important advantages, including access to samples that are several orders of magnitude smaller (from ~gram scales for INS to sub-microgram for IXS), dramatically improved data for disordered materials, as well as generally cleaner data than INS. NRS offers a variety of techniques (see [2,3] and references therein) that probe atomic, magnetic and electronic structure and dynamics on energy scales from neV to meV. While the NRS methods do have some overlap with those of classical Mössbauer spectroscopy (MS), in fact NRS greatly extends the range of available methods, including access to phonon densities of states and to neV-scale atomic dynamics, among others.
The methods employed by the PSD, IXS and NRS, provide detailed and important information that can be difficult or impossible to obtain using other methods and are therefore actively pursued at SPring-8 and, indeed, at most of the large synchrotron radiation facilities including ESRF, APS, PETRA (NRS only) and NSLS-II (IXS only). In this context SPring-8 has the world-leading IXS spectrometers now available[1][1] A. Q. R. Baron: Synch. Light Srcs. & FELS (Springer International Publishing, Cham, 2016) 1643-1757. (DOI: 10.1007/978-3-319-14394-1) See also arXiv 1504.01098. (https://arxiv.org/abs/1504.01098) due in part to a specialized insertion device, and in part to careful design and large analyzer arrays. Meanwhile the NRS program has benefitted from a long tradition of NRS work in Japan[4][4] S. Kikuta, Y. Yoda, K. Izumi, K. Hirano, N. Horiguchi, T. Ishikawa, X. W. Zhang, H. Sugiyama, M. Ando, M. Seto, C. K. Suzuki and S. Nasu: X-Ray Reson. Scatt. (Elsevier, Amsterdam, 1992) 635-646., including a strong focus on instrumentation development[5][5] T. Ishikawa, Y. Yoda, K. Izumi, C. K. Suzuki, X. W. Zhang, M. Ando and S. Kikuta: Rev. Sci. Instrum. 63 (1992) 1015. (DOI: 10.1063/1.1143188).
The PSD is now just completing major changes as the JASRI NRS program moves its main base of operations from BL09XU, where it had been for > 20 years, to BL35XU, as part of the beamline upgrade program. BL35XU had originally been planned for a mixture of IXS and NRS work[6][6] A. Q. R. Baron, Y. Tanaka, S. Goto, K. Takeshita, T. Matsushita and T. Ishikawa: J. Phys. Chem. Solids 61 (2000) 461-465. (DOI: 10.1016/S0022-3697(99)00337-6), but IXS had come to dominate the accepted proposals. The move is indeed a major upgrade for the NRS program, as the specialized insertion device installed at BL35XU provides more than double the flux that was available at BL09XU for most nuclear resonances. However, the move comes with a large reduction (approximately a factor of two) in the beamtime for IXS work at BL35XU. This will be slightly compensated by making some time available for IXS at BL43LXU[7][7] A. Q. R. Baron: SPring-8 Information 15 (2010) 14-19. (https://user.spring8.or.jp/sp8info/?p=3138), somewhat similar to the time made available for NRS at BL19LXU, however, it will nearly certainly be a net loss of time for the IXS program, though the new equilibrium operating point is yet to be established. One hopes to also partly compensate the reduced beamtime with increased efficiency and, perhaps, increased specialization of the IXS program.
2. Nuclear Resonant Scattering (NRS)
The NRS program moved its home base from BL09XU to BL35XU after FY2020 beamtime finished in February of 2021. It continues to also support some experiments at BL19LXU. Commissioning of the NRS setups at BL35XU after the move is now ongoing including beamtime in April, May and July. BL35XU will reopen for general users in October of 2021.
The main changes for NRS at BL35XU relative to BL09XU include
1. Increased flux (~× 2 or more 0).
2. Tuning gap: no flux for energies < 14.4 keV and between 29 and 43 keV.
3. Placement of the high-resolution monochromators (HRMs) in the optics hutch on a table to allow easy switching.
4. Addition of a bent cylindrical mirror that should allow a ~50 micron focal spot below 30 keV and ~150 micron spot size up to ~75 keV.
5. Slightly reduced hutch space.
It is expected that all of the techniques utilized at BL09XU will be available and improved at BL35XU, subject to the caveat that resonances in the ID tuning gap (< 14.4 and 29-43 keV) will not be accessible: for resonances in the gap, time at other beamlines will be needed.
2.1 Optics Hutch: HHLM, HRMs and mirror
The new layout of the optics hutch (OH) is shown in figure 1. The modifications were done by a collaboration of RIKEN and JASRI. The high-heat load monochromator (HHLM) was moved 3.3 m upstream and with the stability and cooling power improved by the optics group.

Figure 1 Overview of the upgraded optics hutch at BL35XU.
A table was added in the OH to allow mounting and easy exchange of the HRMs as the HRMs have a critical role in almost all NRS measurements. Six HRMs for different resonances and/or different resolutions can be set on the optical table. X-stages with a 50 cm stroke allow the HRMs to be easily inserted/removed from the beam path. Available HRMs include nested crystals for 57Fe (14.4 keV) with 2.5 meV & 3.5 meV, 57Fe with 6 meV, 151Eu (21.5 keV), 149Sm (22.5 keV), 119Sn (23.9 keV) which have been used at BL09XU[8][8] Y. Yoda: Hyperfine Interact. 240 (2019) 105. (DOI: 10.1007/s10751-019-1642-3) and the HRM for 161Dy (25.7 keV) developed at BL35XU. For high energy resonances such as 61Ni (67.4 keV) and 174Yb (76.5 keV), a Si 220 channel-cut crystal is also installed on the optical table to reduce harmonics.
A Pt-coated cylindrical mirror (14 mm sagittal radius), similar to BL43LXU, is installed downstream of the HRMs. The sagittal focal length is 3 m at a 2.5 mrad incident angle and the longitudinal focal length is controlled by the bender. This should operate well up to ~30 keV. Focusing at higher energy, to ~75 keV, can be realized by going to smaller grazing angle and relaxing (increasing) the focal length using the flexible bend.
2.2 NRS Spectrometers
There are 3 main techniques using synchrotron radiation for NRS. The spectrometers for these techniques were installed in the experimental hutches: NRS1 and NRS2 as shown in figure 2. The quasi-elastic scattering (QES) spectrometer is placed on the optical table in the NRS2 hutch and composed of the two Mössbauer transducers and He (N2) flow-type cryostat to control the sample temperature preventing vibration, and three APD detectors set at the different Q position. Each APD detector has 2 × 8 elements and all the time spectra are taken by MCS6 and MCS8 produced by Fast ComTec via fast circuits. A pulse-tube cryostat with an APD detector designed for NIS is installed on the same optical table. In case of 57Fe nuclear inelastic scattering (NIS - also sometimes called nuclear resonance vibrational spectroscopy - NRVS), a 0.8 meV resolution HRM may be placed on the optical table in NRS1, as is often used for biological samples. The analyzer for energy domain MS (EDMS) is also placed on the same optical table in the NRS1 hutch. The reference foil in the pulse-tube cryostat is connected to a Mössbauer transducer. The APD detector is set near the foil in vacuum for the analyzer. These spectrometers are installed on optical tables allowing fast switching between the different techniques. The samples for EDMS are placed before the analyzer in the NRS1 hutch. The samples and the APD detector for time domain MS are also placed in the NRS1 hutch. A focused beam is available for 57Fe, 151Eu etc. at around 1.5 m from the upstream wall in the NRS1 hutch. These samples are mounted on the temporally installed optical table or in the super conducting magnet.
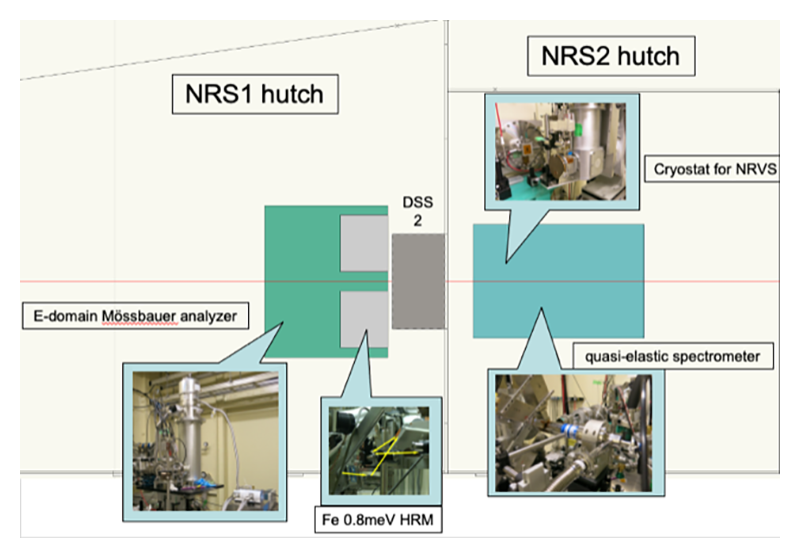
Figure 2 Layout of the NRS hutches at BL35XU.
2.3 Spectral flux
The biggest advantage at BL35XU relative to BL09XU is the higher flux in the energy region popular for NRS due to the short, 20 mm, period undulator at BL35XU. The flux density calculated by SPECTRA is shown in figure 3: more than double the flux is expected, and observed, at 14.4 keV which is a resonant energy of most popular isotope, 57Fe. Higher flux is expected up to the 27.78 keV resonance of 129I. More than double the flux is also expected over 43 keV, including the 174Yb resonance at 76 keV. However, there is a tuning gap (inaccessible region) below 14.4 keV and from 29 keV to 43.2 keV. Therefore, users desiring to work on isotopes in this region such as 169Tm, 83K, 229Th, 40K, 125Te, 121Sb etc. should consider other beamlines. The PSD will support such work, much as we support the NRS work at BL19LXU.
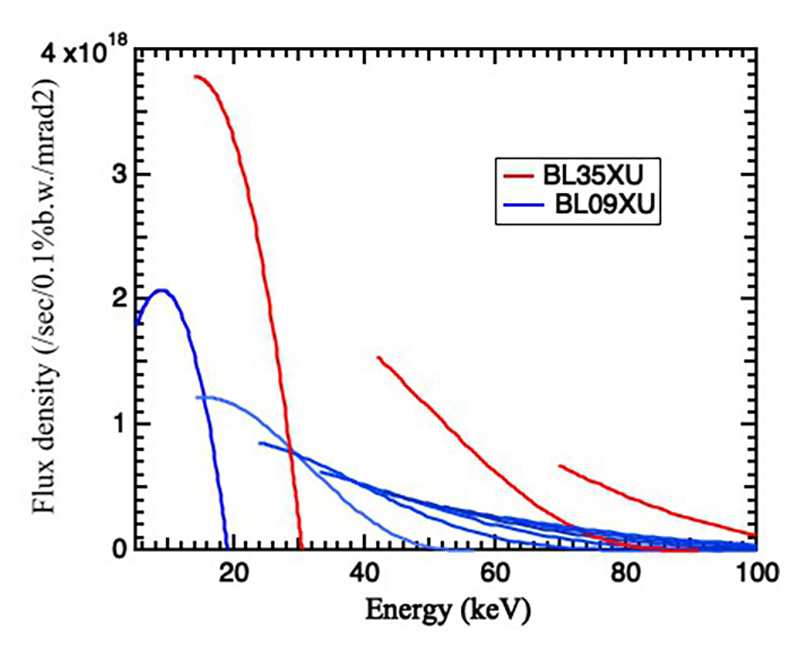
Figure 3 Flux density at BL35XU and BL09XU calculated by SPECTRA.
2.4 NRS Commissioning
Commissioning of NRS at BL35XU is now in progress. For the 57Fe HRMs at 14.4 keV, the focusing mirror and spectrometers for NIS, QES and EDMS were tested in May of 2021, after the commissioning of the HHLM by the optics group. More than double the flux after the HRMs was obtained at all the resolutions. Commissioning using 151Eu, 149Sm, 119Sn, 161Dy and 174Yb is scheduled for July: first tests at the 21.53 keV 151Eu resonance show > 3 × the intensity compared to BL09XU.
3. Inelastic X-Ray Scattering (IXS)
IXS is used to investigate atomic dynamics in materials. The beamline layout is shown in figure 4. There are several standard setups offered at BL35XU[6][6] A. Q. R. Baron, Y. Tanaka, S. Goto, K. Takeshita, T. Matsushita and T. Ishikawa: J. Phys. Chem. Solids 61 (2000) 461-465. (DOI: 10.1016/S0022-3697(99)00337-6) including a > ~1.3 meV resolution setup using the Si(11 11 11) reflection at 21.7 keV, and ~3 meV resolution setup using the Si(999) reflection at 17.8 keV. These can either be used with standard focusing with an ~80 micron beam size or with compound focusing where KB mirrors are used to reduce the size to < 20 microns[9][9] D. Ishikawa, H. Uchiyama, S. Tsutsui, H. Fukui and A. Q. R. Baron: Proc. SPIE 8848 (2013) 88480F. (DOI: 10.1117/12.2023795). The latter is often used with diamond anvil cells (DACs) to investigate materials under extreme pressure. BL35XU also has a setup designed to investigate phonons in thin film samples. The experimental opportunities for IXS at BL43LXU (see [7] and http://beamline.harima.riken.jp/en/bl_info/bl43lxu_info.html) are similar to those at BL35XU, but the flux is higher, with more analyzers and a smaller beam size possible (see also [1]).

Figure 4 Overview of BL35XU-IXS.
The applicable scientific fields for IXS are wide, as phonons and atomic dynamics are relevant for many material properties. Relevant fields include atomic dynamics in liquids and glasses, electron-phonon coupling in correlated materials, including superconductors, sound velocity and elastic constants in extreme conditions (pressures in excess of 300 GPa and temperatures above 3000 K have been achieved in IXS), which are often correlated with seismological work, phonon lifetimes in thermoelectric materials, magneto-elastic coupling, etc. In the following, we provide two examples of recent activity.
The upgrade had nearly no impact on the IXS setups except (1) to improve the stability of the HHLM (a very welcome change after decades of fighting vibrations and drifts) and (2) to reduce the beamtime in 2021. BL35XU shut-down for users in the middle of December of 2020 and was only available for one month in the 2021A round.
3.1 Atomic dynamics in AsSe liquid[10][10] M. Inui, A. Q. R. Baron, Y. Kajihara, K. Matsuda, S. Hosokawa, K. Kimura, Y. Tsuchiya, F. Shimojo, M. Yao, S. Tsutsui, D. Ishikawa and K. Tamura: J. Phys.: Condens. Matter 30 (2018) 28LT02. (DOI: 10.1088/1361-648X/aacab5)
In contrast to inelastic neutron scattering (INS), IXS can easily access atomic dynamics at small momentum transfers. Utilizing this feature, many studies of atomic dynamics in liquids and glasses have been made via IXS. AsxSe1-x is an interesting example, since it is apt to form glass below the melting temperature, especially at x = 0.4. In recent work[10][10] M. Inui, A. Q. R. Baron, Y. Kajihara, K. Matsuda, S. Hosokawa, K. Kimura, Y. Tsuchiya, F. Shimojo, M. Yao, S. Tsutsui, D. Ishikawa and K. Tamura: J. Phys.: Condens. Matter 30 (2018) 28LT02. (DOI: 10.1088/1361-648X/aacab5), IXS experiments were performed in the liquid state (above the melting temperature), in order to address the origin of the glass-forming ability. Given the fact that this liquid has a semiconductor-metal transition at high temperature (> 1300 K) and high pressure (> 5 MPa), the experiments were carried out at temperatures from 1073 to 1673 K at 6 MPa.
Figure 5 shows S(Q,E)/S(Q) of liquid As2Se3 at 1073 and 1473 K. In the upper panel (T = 1073 K), the inelastic component (red) is clearly visible at 2.9 nm-1, while the inelastic component (red) mostly overlaps with the elastic component (blue) at 2.6 nm-1. This indicates a discontinuous jump of the inelastic energy between 2.6 and 2.9 nm-1. In contrast, the spectra in the lower panel (T = 1473 K) do not have a jump: the inelastic energy is mostly proportional to the momentum transfer, as is the normal for the atomic dynamics of liquids. Discontinuous jumps are observed in solid crystals from "anti-crossings" of phonon modes. The observed jump in this liquid may have a similar origin.

Figure 5 S(Q,E)/S(Q) of liquid As2Se3 at 1073 K (upper graph) and 1473 K (lower graph) at 6 MPa.
Further analysis[10][10] M. Inui, A. Q. R. Baron, Y. Kajihara, K. Matsuda, S. Hosokawa, K. Kimura, Y. Tsuchiya, F. Shimojo, M. Yao, S. Tsutsui, D. Ishikawa and K. Tamura: J. Phys.: Condens. Matter 30 (2018) 28LT02. (DOI: 10.1088/1361-648X/aacab5) of AsxSe1-x, was done using rigidity theory, which predicts a stiffness transition from inflexible to flexible structures when the average coordination number falls below 2.4 in the glassy state. For liquid As2Se3, investigation of the local structure reported that the average coordination number of > 2.4 near the melting point decreases with increasing temperature. The model simulations indicate that the disappearance of the discontinuous jump at higher temperatures (≥ 1473 K) originates from the decrease of the average coordination number, and imply existence of a cage structure in the liquid state with the size and lifetime of ~1 nm and ~1 ps at 1373 K.
3.2 Sound velocity of high-pressure iron[11][11] T. Sakamaki, E. Ohtani, H. Fukui, S. Kamada, S. Takahashi, T. Sakairi, A. Takahata, T. Sakai, S. Tsutsui, D. Ishikawa, R. Shiraishi, Y. Seto, T. Tsuchiya and A. Q. R. Baron: Science Adv. 2 (2016) e1500802. (DOI: 10.1126/sciadv.1500802)
The small beam size available for IXS facilitates measurement of samples in extreme conditions, for example, samples in DACs. Since diamond is relatively transparent for hard X-rays and has large sound velocity, atomic dynamics of the samples in DACs can be measured. Furthermore, utilizing a laser heating system where the laser focuses only on the sample in the DAC, measurements in extreme conditions, high temperature and high pressure is possible. In recent work[11][11] T. Sakamaki, E. Ohtani, H. Fukui, S. Kamada, S. Takahashi, T. Sakairi, A. Takahata, T. Sakai, S. Tsutsui, D. Ishikawa, R. Shiraishi, Y. Seto, T. Tsuchiya and A. Q. R. Baron: Science Adv. 2 (2016) e1500802. (DOI: 10.1126/sciadv.1500802), the compressional (longitudinal) sound velocity of hexagonal close-packed (hcp) Fe was measured up to 163 GPa and 3000 K (figure 6) in a laser-heated DAC.

Figure 6 IXS spectrum of hcp-Fe at 163 GPa and 3000 K. (green: elastic scattering, red: LA phonons of hcp-Fe, blue: TA phonons of diamond).
It is well known that the inner core of the Earth mainly consists of Fe, in the form of hcp-Fe. However, earth-scientists greatly desire detailed information of the remaining minor components. Results from the seismological data have been interpreted in using the Preliminary Reference Earth Model (PREM) and suggest the minor components in the inner core are light elements, such as silicon, sulfur, oxygen, hydrogen, and carbon, since the density of inner core is about 2-5% less than that of hcp-Fe.
IXS measurements[10][10] M. Inui, A. Q. R. Baron, Y. Kajihara, K. Matsuda, S. Hosokawa, K. Kimura, Y. Tsuchiya, F. Shimojo, M. Yao, S. Tsutsui, D. Ishikawa and K. Tamura: J. Phys.: Condens. Matter 30 (2018) 28LT02. (DOI: 10.1088/1361-648X/aacab5), showed the compressional sound velocity of hcp-Fe under the inner core condition was ~7% larger than the velocity predicted in PREM. The results indicate that the light elements or the combination of the light elements and nickel reduces both the density and sound velocity of hcp-Fe in the inner core. Furthermore, from comparison with other experimental data, silicon, sulfur, and hydrogen are potential candidates as light elements because these reduces both the density and velocity.
4. Concluding Comments
The Precision Spectroscopy Division is beginning to establish a new equilibrium after significant changes in both beamline operations and in organization. The preliminary indications are that the beamline upgrade has significantly improved NRS capability at SPring-8: the observed increased flux will allow major gains for NRS experiments as they are almost all count-rate limited. There are also plans for stabilization of some setups and some specific upgrades to instrumentation. The NRS upgrade, however, unfortunately comes at the cost of a major reduction in the available beamtime for IXS: we are partly compensating for that with some time at other beamlines, and also hope to improve efficiency, possibly by increasing specialization. Another big change on the ground in the PSD is the increase in scientific staff, with the recent hire (April 2021) of a new tenure-track researcher in NRS significantly increasing manpower for that work. Meanwhile, the planned hire of a one more tenure track researcher focused on IXS is both expected to help improve efficiency and hopefully allow expansion in some new directions.
References
[1] A. Q. R. Baron: Synch. Light Srcs. & FELS (Springer International Publishing, Cham, 2016) 1643-1757. (DOI: 10.1007/978-3-319-14394-1) See also arXiv 1504.01098. (https://arxiv.org/abs/1504.01098)
[2] E. Gerdau and H. de Waard: Hyperfine Interact. 123-125 (1999-2000).
[3] M. Seto: J. Phys. Soc. Japan 82 (2013) 021016. (DOI: 10.7566/JPSJ.82.021016)
[4] S. Kikuta, Y. Yoda, K. Izumi, K. Hirano, N. Horiguchi, T. Ishikawa, X. W. Zhang, H. Sugiyama, M. Ando, M. Seto, C. K. Suzuki and S. Nasu: X-Ray Reson. Scatt. (Elsevier, Amsterdam, 1992) 635-646.
[5] T. Ishikawa, Y. Yoda, K. Izumi, C. K. Suzuki, X. W. Zhang, M. Ando and S. Kikuta: Rev. Sci. Instrum. 63 (1992) 1015. (DOI: 10.1063/1.1143188)
[6] A. Q. R. Baron, Y. Tanaka, S. Goto, K. Takeshita, T. Matsushita and T. Ishikawa: J. Phys. Chem. Solids 61 (2000) 461-465. (DOI: 10.1016/S0022-3697(99)00337-6)
[7] A. Q. R. Baron: SPring-8 Information 15 (2010) 14-19. (https://user.spring8.or.jp/sp8info/?p=3138)
[8] Y. Yoda: Hyperfine Interact. 240 (2019) 105. (DOI: 10.1007/s10751-019-1642-3)
[9] D. Ishikawa, H. Uchiyama, S. Tsutsui, H. Fukui and A. Q. R. Baron: Proc. SPIE 8848 (2013) 88480F. (DOI: 10.1117/12.2023795)
[10] M. Inui, A. Q. R. Baron, Y. Kajihara, K. Matsuda, S. Hosokawa, K. Kimura, Y. Tsuchiya, F. Shimojo, M. Yao, S. Tsutsui, D. Ishikawa and K. Tamura: J. Phys.: Condens. Matter 30 (2018) 28LT02. (DOI: 10.1088/1361-648X/aacab5)
[11] T. Sakamaki, E. Ohtani, H. Fukui, S. Kamada, S. Takahashi, T. Sakairi, A. Takahata, T. Sakai, S. Tsutsui, D. Ishikawa, R. Shiraishi, Y. Seto, T. Tsuchiya and A. Q. R. Baron: Science Adv. 2 (2016) e1500802. (DOI: 10.1126/sciadv.1500802)
(公財)高輝度光科学研究センター
放射光利用研究基盤センター 精密分光推進室
〒679-5198 兵庫県佐用郡佐用町光都1-1-1
TEL : 0791-58-0802
e-mail : yoda@spring8.or.jp
(公財)高輝度光科学研究センター
放射光利用研究基盤センター 精密分光推進室
〒679-5198 兵庫県佐用郡佐用町光都1-1-1
TEL : 0791-58-0802
e-mail : uchiyama@spring8.or.jp
(公財)高輝度光科学研究センター
放射光利用研究基盤センター 精密分光推進室
〒679-5198 兵庫県佐用郡佐用町光都1-1-1
TEL : 0791-58-0802
e-mail : disikawa@spring8.or.jp
(公財)高輝度光科学研究センター
放射光利用研究基盤センター 精密分光推進室
〒679-5198 兵庫県佐用郡佐用町光都1-1-1
TEL : 0791-58-0802
e-mail : nagasawa@spring8.or.jp
(公財)高輝度光科学研究センター
放射光利用研究基盤センター 精密分光推進室
〒679-5198 兵庫県佐用郡佐用町光都1-1-1
TEL : 0791-58-0802
e-mail : baron@spring8.or.jp