Volume 17, No.1 Pages 26 - 32
1. 最近の研究から/FROM LATEST RESEARCH
Long-term Proposal Report:
Spin-resolved Hard X-ray Photoelectron Spectroscopy Spin-HAXPES
[1] Institut für Anorganische und Analytische Chemie, Johannes Gutenberg - Universität Mainz、 [2] Max-Planck-Institut für Chemische Physik fester Stoffe、 [3] Institut für Physik, Johannes Gutenberg - Universität Mainz、 [4] Research & Utilization Division, JASRI、 [5] Division of Electronics for Informatics, Hokkaido University、 [6] Magnetic Materials Center, NIMS、 [7] NIMS Beamline Station at SPring-8, NIMS、 [8] Hiroshima Synchrotron Radiation Center, Hiroshima University
- Abstract
- The spin-resolved electronic structure of buried magnetic layers is studied by hard X-ray photoelectron spectroscopy (HAXPES) using a spin polarimeter in combination with a high-energy hemispherical electron analyzer at the high-brilliance BL47XU beamline. Spin-resolved photoelectron spectra are analyzed in comparison with the results of magnetic linear and circular dichroism in photoelectron emission in the case of buried Co2FeAl0.5Si0.5 layers. The relatively large inelastic mean free path (up to 20 nm) of fast photoelectrons enables us to extend the HAXPES technique with electron-spin polarimetry and to develop spin analysis techniques for buried magnetic multilayers and interfaces.
1. Introduction
The spin-resolved exploration of the electronic structure of buried ferromagnetic layers and interfaces is of great importance. It is needed for the selection of optimal materials for spintronic devices. For example, the efficiency of magnetic tunnel junctions (MTJs) is largely defined by the spin-dependent electronic properties of half-metallic electrode materials [1, 2][1]J. Kübler, A. R. Williams and C. B. Sommers: Phys. Rev. B 28 (1983) 1745.
[2]R. A. de Groot, F. M. Müller, P. G. van Engen and K. H. J. Buschow: Phys. Rev. Lett. 50 (1983) 2024. employed.
Hard X-ray photoelectron spectroscopy (HAXPES) was proved to be a bulk sensitive probe of the electronic band structure [3, 4][3]K. Kobayashi, M. Yabashi, Y. Takata, T. Tokushima, S. Shin, K. Tamasaku, D. Miwa, T. Ishikawa, H. Nohira, T. Hattori, Y. Sugita, O. Nakatsuka, A. Sakai and S. Zaima: Appl. Phys. Lett. 83 (2003) 1005.
[4]G. H. Fecher, B. Balke, A. Gloskowskii, S. Ouardi, C. Felser, T. Ishikawa, M. Yamamoto, Y. Yamashita, H. Yoshikawa, S. Ueda and K. Kobayashi: Appl. Phys. Lett. 92 (2008) 193513.. The high bulk sensitivity of this technique results from the large inelastic mean free path (10-20 nm) of high-energy photoelectrons (6-10 keV). Such a high bulk sensitivity enables us to explore the electronic band structure of buried layers and interfaces. In this work, we present the results of the extension of a HAXPES experiment with a spin polarimeter implemented at the BL47XU beamline [5][5]G. Stryganyuk, X. Kozina, G. H. Fecher, S. Ouardi, S. Chadov, C. Felser, G. Schoenhense, A. Oelsner, P. Bernhard, E. Ikenaga, T. Sugiyama, H. Sukegawa, Z. Wen, K. Inomata and K. Kobayashi: Jpn. J. Appl. Phys. 51 (2012) 016602.. This combination facilitates complete studies of the electronic band structure on buried layers resolving electron energy, momentum, and spin degrees of freedom.
Spin-resolved photoelectron emission studies performed in the Ultraviolet and soft X-ray modes of Photoelectron Spectroscopy (UPS/XPS) imply a high surface sensitivity due to the small inelastic mean free path of photoelectrons with kinetic energies in the range <2 keV. The implementation of spin detection in a bulk-sensitive spin-resolved HAXPES experiment implies rather strong requirements on the electron focusing system and conditions of high potential within a spin detecting system. This is crucial in the case of low-energy electron scattering in electron-scattering-type spin analyzers. Photoelectron spin polarization analysis is hampered by a strong reduction of intensity at the spin-discriminating stage by 3-4 orders of magnitude. In combination with the low photoionization cross sections at high kinetic energies [3, 6, 7][3]K. Kobayashi, M. Yabashi, Y. Takata, T. Tokushima, S. Shin, K. Tamasaku, D. Miwa, T. Ishikawa, H. Nohira, T. Hattori, Y. Sugita, O. Nakatsuka, A. Sakai and S. Zaima: Appl. Phys. Lett. 83 (2003) 1005.
[6]G. H. Fecher, B. Balke, S. Ouardi, C. Felser, G. Schönhense, E. Ikenaga, J.-J. Kim, S. Ueda and K. Kobayashi: J. Phys. D 40 (2007) 1576.
[7]K. Kobayashi: Nucl. Instrum. Methods Phys. Res., Sect. A 601 (2009) 32., this leads to low count rates.
Presently available spin detection techniques are based on the spin dependence in electron scattering and diffraction originating from spin-orbit interaction or exchange scattering. The error of spin-polarization measurements and their efficiency depend on the electron reflectivity at the spin-discriminating target surface and spin-resolving power S (Sherman function) defining the figure of merit of the employed electron spin detector. Widely used Mott detectors [8][8]T. J. Gay and F. B. Dunning: Rev. Sci. Instrum. 63 (1992) 1635. suffer from a low figure of merit (FoM of 10-4 order), which is improved up to 10-3 order in the case of SPLEED-type detectors [9][9]D. Yu, C. Math, M. Meier, M. Escher, G. Rangelov and M. Donath: Surf. Science 601 (2007) 5803. based on the Spin-Polarized Low Energy (about 100 eV) Electron Diffraction. Rather efficient are the spin detectors based on the spin-dependent reflection (exchange scattering) of very low energy electrons (scattering energy of about 6-15 eV) from magnetized surfaces [10][10]A. Winkelmann, D. Hartung, H. Engelhard, C.-T. Chiang and J. Kirschner: Rev. Sci. Instrum. 79 (2008) 083303. with the reported FoM of 10-2 order [11][11]T. Okuda, Y. Takeichi, Y. Maeda, A Harasawa, I. Matsuda, T. Kinoshita and A. Kakizaki: Rev. Sci. Instrum. 79 (2008) 123117.. Prospective breakthrough in the spin-resolved photoelectron spectroscopy is expected from the recently suggested multichannel spin-filtering technique [12][12]M. Kolbe, P. Lushchyk, B. Petereit, H. J. Elmers, G. Schoenhense, A. Oelsner, C. Tusche and J. Kirschner: Phys. Rev. Lett. 107 (2011) 207601. with the FoM of 2.1 achieved.
Related information on the majority and minority spin characters of the electronic states may also be obtained in magnetic linear and circular dichroisms in X-ray absorption [13][13]P. Carra and M. Altarelli: Phys. Rev. Lett. 64 (1990) 1286. and photoelectron emission [14, 15][14]L. Baumgarten, C. M. Schneider, H. Petersen, F. Schaefers and J. Kirschner: Phys. Rev. Lett. 65 (1990) 492.
[15]G. van der Laan: Phys. Rev. B 51 (1995) 240.. Magnetic dichroism phenomena in photoelectron emission exemplify a preferential interaction of polarized X-rays with electrons of a particular spin direction defined by the experimental geometry. They enable us to elucidate spin splitting in core multiplet states [16][16]C. S. Fadley and D. A. Shierley: Phys. Rev. A 2 (1970) 1109. due to the exchange interaction of the core hole with the spin-polarized bands of valence electrons. The present studies involve a comparative analysis of spin-resolved photoelectron spectra together with data on magnetic circular and linear dichroisms in angular distribution (MCDAD, MLDAD) in photoelectron emission.
2. Experimental Procedure
The spin-resolved HAXPES experiment has been implemented by a combination of a Scienta R-4000-10 keV hemispherical analyzer with a spin detector based on Spin-Polarized Low-Energy Electron Diffraction (SPLEED) at a W(100) surface [17][17]D. Yu, C. Math, M. Meier, M. Escher, G. Rangelov and M. Donath: Surf. Sci. 601 (2007) 5803.. For the spin-resolved measurements with the SPLEED-type detector, the photon energy was set to 5.95 keV because of the higher photoelectron intensity expected from the increase of ionization cross section at lower energies. The photon flux at 5.95 keV was about 1011 photons/s with a 50 meV intrinsic bandwidth after a Si(333) channel cut post-monochromator [7][7]K. Kobayashi: Nucl. Instrum. Methods Phys. Res., Sect. A 601 (2009) 32.. The total energy resolution for spin-resolved measurements was set to about 1 eV with a fixed 2 mm entrance slit of the SPLEED detector and a 200 eV pass energy of the analyzer.
The angle between the electron analyzer and the direction of photon incidence was fixed at 90 deg. The photoelectrons were collected from the sample in near-normal emission geometry. Linearly polarized photons of Pp=0.99 polarization degree were obtained from the undulator without any polarizing optics. The electric E vector of photons in this case was oriented in the plane of beam incidence (p-polarization), i.e., near perpendicular to the sample surface in the near-normal emission geometry used.
Studies of magnetic dichroism were performed with 7.94 keV photons. An in-vacuum phase retarder employing a 600 μm thick diamond (220) crystal [18, 19][18]M. Suzuki, N. Kawamura, M. Mizukami, A. Urata, H. Maruyama, S. Goto and T. Ishikawa: Jpn. J. Appl. Phys. 37 (1998) L1488.
[19]S. Ouardi, G. H. Fecher, X. Kozina, G. Stryganyuk, B. Balke, C. Felser, E. Ikenaga, T. Sugiyama, N. Kawamura, M. Suzuki and K. Kobayashi: Phys. Rev. Lett. 107 (2011) 036402. has been installed at the BL47XU beamline in order to vary the polarization of the 7.94 keV photon beam. Polarization degrees of Pc=0.95 and Ps=0.90 have been reached for circularly polarized and linearly s-polarized photons, respectively. The photon flux at 7.94 keV was ≈4×1011 photons/s with a bandwidth of 38 meV achieved with a Si(111) double crystal monochromator and a Si(444) channel cut post-monochromator [7][7]K. Kobayashi: Nucl. Instrum. Methods Phys. Res., Sect. A 601 (2009) 32.. The total energy resolution for magnetic dichroism studies was set to 150 meV.
An experiment was performed on exchange-biased Co2FeAl0.5Si0.5 (CFAS) thin films shown to be a half-metallic Heusler compound with a high spin polarization exhibiting the lowest temperature dependence among the known half-metals [20][20]R. Shan, H. Sukegawa, W. H. Wang et al.: Phys. Rev. Lett. 102 (2009) 246601..
CoFe (10 nm)/Ir22Mn78 (10 nm)/CoFe (0.5 nm)/CFAS (15 nm)/MgO (2 nm)/AlOx (1 nm) multilayers were deposited on a MgO(100) substrate. An exchange bias field of 12 kA m-1 was achieved for the in-plane magnetization after the in-vacuum annealing of a multilayer stack at 300°C followed by cooling in a magnetic field of 400 kA m-1.
The spin-polarized electronic states of buried magnetic layers were studied with special emphasis on the bulk sensitivity of HAXPES and the correlation of the spin-resolved photoelectron spectra with magnetic linear and circular dichroism in photoelectron emission from a CFAS layer buried under a 3 nm thick protective coating. The results are discussed in terms of a multiplet model, explaining the magnetic dichroism in photoelectron emission.
3. Results and Discussion
Figure 1 shows the spin-resolved photoelectron spectra of the Fe 2p3/2 state upon excitation with p-polarized 5.95 keV photons. A maximum polarization of about 50% is obtained after background subtraction according to the following equation:
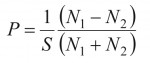
with N1,2 denoting the countrates in the spin detector channels and with the Sherman function S being set to 0.25 [17][17]D. Yu, C. Math, M. Meier, M. Escher, G. Rangelov and M. Donath: Surf. Sci. 601 (2007) 5803.. The majority and minority spin partial spectra were determined as
I↑,↓ = Itotal (1 ± P)/2. (2)
For details on the spin polarization detection see [21, 22][21]J. Kessler. in Polarized Electrons, ed. G. Ecker, P. Lambropoulos and H. Walther (Springer, Berlin, 1985): Springer Series on Atoms and Plasmas, Vol. 1, p. 299.
[22]J. Kirschner. in Polarized Electrons at Surfaces, ed. G. Hohler (Springer, Berlin, 1985): Springer Acts in Modern Physics, Vol. 106, p. 155..
Count rates in spin detector channels (a), spin polarization (b) and spin-resolved spectra of Fe 2p3/2 photoelectrons (c) from buried CFAS layer. The energy resolution interval (1 eV) is shown with a vertical slit mark.
The structure of the Fe 2p3/2 mj multiplet (shown as bars in Fig. 1c) was taken from the band structure calculation of Co2FeAl0.5Si0.5 performed by the Spin-Polarized fully Relativistic method of Korringa-Kohn-Rostoker (KKR) Green’s function (SPR-KKR) [23][23]H. Ebert and M. Battocletti: Solid State Commun. 98 (1996) 785.. The bars topped with filled and hollow triangles depict the states with negative and positive spin projection ms values; the larger and smaller bars correspond to the |mj|=3/2 and |mj|=1/2 states, respectively. The sub-states of the 2p3/2 core electrons with well-defined spin up (mj=+3/2; ms=+1/2) and spin down (mj=-3/2; ms=-1/2) are clearly reproduced by maxima in the spin-resolved photoelectron spectra (Fig. 1c). The electron spin (ms) is not well defined for 2p3/2 states with mj=±1/2 since the respective mj values (mj=ml+ms) may be obtained with different ml and ms combinations. The 2p3/2 state with mj=-1/2 is, however, revealed as a shoulder in the spin down component. Thus, the features of Fe 2p3/2 core states are well reproduced in the measured spin-resolved phototelectron spectra showing a good agreement with the one-step model of photoelectron emission.
One should note the broadening of the measured spin-resolved photoelectron spectra. This broadening may originate from a relatively poor resolution of the spin-resolved measurements, but this is not the only reason. After electron escape from the Fe 2p orbital, the coupling of the 2p5 (2P3/2) core hole with 3d5 (6S5/2) valence electrons results in a variety of ionic 7,5P|L+S|≈J≈|L-S| configurations (see [24][24]X. Kozina, G. H. Fecher, G. Stryganyuk, S. Ouardi, B. Balke, C. Felser, G. Schoenhense, E. Ikenaga, T. Sugiyama, N. Kawamura, M. Suzuki, T. Taira, T. Uemura, M. Yamamoto, H. Sukegawa, W. Wang, K. Inomata and K. Kobayashi: Phys. Rev. B 84 (2011) 054449. for details). The difference in the energy levels of the ionic states implies a broad kinetic energy distribution for the emitted electrons and the broadening of the photoemission spectra, particularly at the side of higher binding energies [25][25]F. de Groot and A. Kotani: Core Level Spectroscopy of Solids, ed. D. D. Sarma, G. Kotliar and Y. Tokura: (CRC Press, Boca Raton, FL, 2008) Advances in Condensed Matter Science, Vol. 6, p. 490..
The observed spin polarization of photoelectrons from a core state may be considered as an indirect evidence for the spin-polarized valence states. It arises due to the presence of a strong exchange field (Weiss field) in the material. The spin polarization at the Fermi level can only be elucidated from a spin-resolved measurement of the valence states. However, the density of valence states is reduced by about one order of magnitude as compared with that of the core states. Therefore, the spin-HAXPES experiment on the valence states has been planned as a further step forward after commissioning the modified SPLEED-type spin detector with an improved transmission efficiency of its electron focusing system and we are going to implement also the novel multichannel concept of electron spin detection [26][26]M. Kolbe, P. Lushchyk, B. Petereit, H. J. Elmers, G. Schoenhense, A. Oelsner, C. Tusche and J. Kirschner: Phys. Rev. Lett. 107 (2011) 207601. in HAXPES mode.
Figure 2 shows the correlation between spin-resolved photoelectron spectra and magnetic circular dichroism (MCDAD) as well as magnetic linear dichroisms. MCDAD in photoelectron emission (acceptance angle of ±7 deg. tilted by 2 deg. with respect to the sample surface normal was measured with the helicity vector σ of circularly polarized 7.94 keV photons directed nearly parallel (σ+) and antiparallel (σ-) to the magnetization vector M of the in-plane magnetized sample. A maximal MCDAD value of 45% was reached for the Fe 2p3/2 photoelectrons from the CFAS layer.
Comparison of spin-resolved Fe 2p3/2 spectra with MCDAD and MLDAD for photoelectron from buried CFAS layer. The corresponding energy resolution intervals are shown by vertical slit marks.
The 1.3 eV gap between the maxima of the spin-resolved photoelectron spectra is in good agreement with the exchange splitting revealed by MCDAD measurements. Such a relatively large exchange splitting proves the magnetic moment to be localized at Fe sites in CFAS. The extrema of the MCDAD signal show a slight shift to higher binding energies as compared with the maxima of the spin-resolved peaks.
Having the photoelectron spin-resolved spectrum and magnetic circular dichroism measured for a core state, one can estimate the spin and orbital moments using the sum-rules-type procedure suggested by Thole and van der Laan [27][27]B. T. Thole and G. van der Laan: Phys. Rev. B 50 (1994) 11474.. However, this procedure implies the calculations of the spin-resolved core level photoelectron spectra measured for both spin-orbit components upon excitation with isotropic light (spherically polarized photons). Such experiment can be implemented upon the excitation with an incoherent superposition of two normally oriented beams of unpolarized and linearly polarized photons.
Magnetic linear dichroism (MLDAD) was measured with s-polarized photons (7.94 keV) and the sample magnetization along or perpendicular to the E vector of the X-rays. The MLDAD signal changes its sign at approximately 706.8 eV (Fig.2b) where the "minority" signal in the spin-resolved photoelectron spectra starts to increase. The MLDAD shows a well pronounced negative asymmetry with a 12% amplitude in the range of the Fe 2p3/2 state with the spin of the minority sign. The higher probability for the emission of the Fe 2p electron with a minority spin (as it is recognized for M || E, Fig. 2c) is caused by the higher probability for the coupling of the Fe 2p5 hole with Fe 3d5 electrons resulting in the state with a maximal majority spin. Thus, the magnetic dichroism in photoelectron emission elucidates the spin-polarized electronic states and enables us to recognize the spin sign relatively to the majority d states of the valence band. MLDAD measurements show a higher intensity for the electrons with a minority spin when the magnetization vector M is aligned parallel to the E vector of the photons.
Conclusions
In summary, a spin-resolved HAXPES experiment was successfully performed on a buried magnetic layer. The implemented Spin-HAXPES technique facilitates the direct observation of the spin polarization of emitted electrons. The measurements prove that a spin polarization of about 50% is retained during the transmission of photoelectrons emitted from the Fe 2p3/2 state through a 3 nm thick oxide capping layer. The reported spin-resolved photoelectron spectra agree well with the magnetic circular and linear dichroisms. The informations from spin polarization and magnetic dichroism are different and their combination provides a detailed insight into the dynamics of photoemission from a ferromagnetic material. The reported experiment paves the way to the realization of the spin-resolved spectroscopy of buried layers and buried interfaces, one of the issues inaccessible by the classical low-energy approach.
The magnetic states can be elucidated from magnetic dichroism measurements providing much higher detection efficiency [24][24]X. Kozina, G. H. Fecher, G. Stryganyuk, S. Ouardi, B. Balke, C. Felser, G. Schoenhense, E. Ikenaga, T. Sugiyama, N. Kawamura, M. Suzuki, T. Taira, T. Uemura, M. Yamamoto, H. Sukegawa, W. Wang, K. Inomata and K. Kobayashi: Phys. Rev. B 84 (2011) 054449. than that achieved in spin-resolved measurements with a spin detector. However, this does not exclude the necessity in direct spin-resolved measurements with spin detectors since the technique of spin-polarized state restoration from MCDAD and MLDAD is not well established yet.
Thus, spin-resolution in the HAXPES of valence states is highly desirable because it will provide the true electronic band structure of a bulk material. In particular, it avoids the various problems caused by surface states, surface reconstruction, and its termination or contamination.
Acknowledgments
Financial support by Deutsche Forschungsgemeinschaft and the Strategic International Cooperative Program of JST is gratefully acknowledged (DFG-JST: FE633/6-1). The synchrotron radiation measurements were performed at BL47XU with the approval of the Japan Synchrotron Radiation Research Institute (JASRI; 2009B0017).
References
[1]J. Kübler, A. R. Williams and C. B. Sommers: Phys. Rev. B 28 (1983) 1745.
[2]R. A. de Groot, F. M. Müller, P. G. van Engen and K. H. J. Buschow: Phys. Rev. Lett. 50 (1983) 2024.
[3]K. Kobayashi, M. Yabashi, Y. Takata, T. Tokushima, S. Shin, K. Tamasaku, D. Miwa, T. Ishikawa, H. Nohira, T. Hattori, Y. Sugita, O. Nakatsuka, A. Sakai and S. Zaima: Appl. Phys. Lett. 83 (2003) 1005.
[4]G. H. Fecher, B. Balke, A. Gloskowskii, S. Ouardi, C. Felser, T. Ishikawa, M. Yamamoto, Y. Yamashita, H. Yoshikawa, S. Ueda and K. Kobayashi: Appl. Phys. Lett. 92 (2008) 193513.
[5]G. Stryganyuk, X. Kozina, G. H. Fecher, S. Ouardi, S. Chadov, C. Felser, G. Schoenhense, A. Oelsner, P. Bernhard, E. Ikenaga, T. Sugiyama, H. Sukegawa, Z. Wen, K. Inomata and K. Kobayashi: Jpn. J. Appl. Phys. 51 (2012) 016602.
[6]G. H. Fecher, B. Balke, S. Ouardi, C. Felser, G. Schönhense, E. Ikenaga, J.-J. Kim, S. Ueda and K. Kobayashi: J. Phys. D 40 (2007) 1576.
[7]K. Kobayashi: Nucl. Instrum. Methods Phys. Res., Sect. A 601 (2009) 32.
[8]T. J. Gay and F. B. Dunning: Rev. Sci. Instrum. 63 (1992) 1635.
[9]D. Yu, C. Math, M. Meier, M. Escher, G. Rangelov and M. Donath: Surf. Science 601 (2007) 5803.
[10]A. Winkelmann, D. Hartung, H. Engelhard, C.-T. Chiang and J. Kirschner: Rev. Sci. Instrum. 79 (2008) 083303.
[11]T. Okuda, Y. Takeichi, Y. Maeda, A Harasawa, I. Matsuda, T. Kinoshita and A. Kakizaki: Rev. Sci. Instrum. 79 (2008) 123117.
[12]M. Kolbe, P. Lushchyk, B. Petereit, H. J. Elmers, G. Schoenhense, A. Oelsner, C. Tusche and J. Kirschner: Phys. Rev. Lett. 107 (2011) 207601.
[13]P. Carra and M. Altarelli: Phys. Rev. Lett. 64 (1990) 1286.
[14]L. Baumgarten, C. M. Schneider, H. Petersen, F. Schaefers and J. Kirschner: Phys. Rev. Lett. 65 (1990) 492.
[15]G. van der Laan: Phys. Rev. B 51 (1995) 240.
[16]C. S. Fadley and D. A. Shierley: Phys. Rev. A 2 (1970) 1109.
[17]D. Yu, C. Math, M. Meier, M. Escher, G. Rangelov and M. Donath: Surf. Sci. 601 (2007) 5803.
[18]M. Suzuki, N. Kawamura, M. Mizukami, A. Urata, H. Maruyama, S. Goto and T. Ishikawa: Jpn. J. Appl. Phys. 37 (1998) L1488.
[19]S. Ouardi, G. H. Fecher, X. Kozina, G. Stryganyuk, B. Balke, C. Felser, E. Ikenaga, T. Sugiyama, N. Kawamura, M. Suzuki and K. Kobayashi: Phys. Rev. Lett. 107 (2011) 036402.
[20]R. Shan, H. Sukegawa, W. H. Wang et al.: Phys. Rev. Lett. 102 (2009) 246601.
[21]J. Kessler. in Polarized Electrons, ed. G. Ecker, P. Lambropoulos and H. Walther (Springer, Berlin, 1985): Springer Series on Atoms and Plasmas, Vol. 1, p. 299.
[22]J. Kirschner. in Polarized Electrons at Surfaces, ed. G. Hohler (Springer, Berlin, 1985): Springer Acts in Modern Physics, Vol. 106, p. 155.
[23]H. Ebert and M. Battocletti: Solid State Commun. 98 (1996) 785.
[24]X. Kozina, G. H. Fecher, G. Stryganyuk, S. Ouardi, B. Balke, C. Felser, G. Schoenhense, E. Ikenaga, T. Sugiyama, N. Kawamura, M. Suzuki, T. Taira, T. Uemura, M. Yamamoto, H. Sukegawa, W. Wang, K. Inomata and K. Kobayashi: Phys. Rev. B 84 (2011) 054449.
[25]F. de Groot and A. Kotani: Core Level Spectroscopy of Solids, ed. D. D. Sarma, G. Kotliar and Y. Tokura: (CRC Press, Boca Raton, FL, 2008) Advances in Condensed Matter Science, Vol. 6, p. 490.
[26]M. Kolbe, P. Lushchyk, B. Petereit, H. J. Elmers, G. Schoenhense, A. Oelsner, C. Tusche and J. Kirschner: Phys. Rev. Lett. 107 (2011) 207601.
[27]B. T. Thole and G. van der Laan: Phys. Rev. B 50 (1994) 11474.
Gregory STRYGANYUK
Johannes Gutenberg - Universität Mainz
Institut für Anorganische und Analytische Chemie
〒55099 Mainz, Germany
TEL:0049 6131 39 22488
e-mail:stryganyuk@gmail.com
Xeniya KOZINA
Johannes Gutenberg - Universität Mainz
Institut für Anorganische und Analytische Chemie
〒55099 Mainz, Germany
TEL:0049 6131 39 23222
e-mail:kozina@uni-mainz.de
Gerhard H. FECHER
Max-Planck-Institut für Chemische Physik fester Stoffe
〒1187 Nöthnitzer Straße 40, Dresden, Germany
TEL:0049 351 4646 3001
e-mail:fecher@cpfs.mpg.de
Johannes Gutenberg - Universität Mainz
Institut für Anorganische und Analytische Chemie
〒55099 Mainz, Germany
TEL:0049 6131 39 25613
e-mail:fecher@uni-mainz.de
Claudia FELSER
Max-Planck-Institut für Chemische Physik fester Stoffe
〒1187 Nöthnitzer Straße 40, Dresden, Germany
TEL:0049 351 4646 3001
e-mail:felser@cpfs.mpg.de
Johannes Gutenberg - Universität Mainz
Institut für Anorganische und Analytische Chemie
〒55099 Mainz, Germany
TEL:0049 6131 39 26266
e-mail:felser@uni-mainz.de
Gerd SCHÖNHENSE
Johannes Gutenberg - Universität Mainz
Institut für Physik
〒55099 Mainz, Germany
TEL:0049 6131 39 22276
e-mail:schoenhe@uni-mainz.de
Eiji IKENAGA
Japan Synchrotron Radiation Research Institute
Research & Utilization Division
〒679-5198 1-1-1 Kouto, Sayo, Hyogo, Japan
TEL:0081 791 58 0802
e-mail:ikenaga@spring8.or.jp
Takeharu SUGIYAMA
Japan Synchrotron Radiation Research Institute
Research & Utilization Division
〒679-5198 1-1-1 Kouto Sayo, Hyogo, Japan
TEL:0081 791 58 0833
e-mail:sugiyama@spring8.or.jp
Masafumi YAMAMOTO
Hokkaido University
Division of Electronics for Informatics
〒060-0814 Kita 14, Nishi 9, Kita-ku, Sapporo, Japan
TEL:0081 11 706 6439
e-mail:yamamoto@nano.ist.hokudai.ac.jp
Koichiro INOMATA
National Institute for Material Science
Magnetic Materials Center
〒305-0047 1-2-1 Sengen, Tsukuba, Ibaraki, Japan
TEL:0081 29 859 2540
e-mail:INOMATA.Kouichiro@nims.go.jp
Keisuke KOBAYASHI
National Institute for Material Science
NIMS Beamline Station at SPring-8
〒679-5148 1-1-1 Kouto, Sayo, Hyogo, Japan
TEL:0081 791 58 1970
e-mail:koba_kei@spring8.or.jp
Hiroshima University
Hiroshima Synchrotron Radiation Center, HiSOR
〒739-0046 Higashihiroshima, Hiroshima, Japan