Volume 26, No.4 Pages 372 - 376
1. 最近の研究から/FROM LATEST RESEARCH
Long-term Proposal Report 2
Identifying Mechanisms to Improve Newborn Respiratory Function using Phase Contrast X-ray Imaging
[1]The Ritchie Centre, Hudson Institute of Medical Research / Department of Obstetrics and Gynaecology, Monash University、[2]School of Physics and Astronomy, Monash University
- Abstract
- The clearance of airway liquid at birth represents a major challenge for newborn infants and must be accomplished within a few minutes of birth to allow the onset of pulmonary gas exchange. While the majority of infants perform this task with remarkable ease, up to 20% of infants require some sort of assistance to make this transition. Phase contrast X-ray imaging studies at SPring-8 have uncovered some of the mysteries behind this process, showing that liquid is cleared from the airways into lung tissue due to pressure gradients generated by inspiration or positive pressure inflations. While the newborn is well adapted to cope with the resulting lung oedema, its capacity to do so is limited. Thus, when airway liquid volumes are high at the onset of air breathing the infant can suffer respiratory distress after birth, which usually develops within 1-2 hours of birth and is 4 times more likely to occur in infants born by caesarean section at or near term. Subsequent studies at SPring-8 have now explained the pathophysiology associated with this form of respiratory distress and shown that it arises from the newborn having a larger than normal volume of liquid present within the airways at the onset of air-breathing. As a result, larger than normal volumes of liquid must be accommodated within lung tissue following lung aeration, which causes excessive pulmonary oedema; the larger the volume, the greater the oedema. This type of pathophysiology is very similar to the pulmonary pathophysiology suffered by patients with a severe COVID-19 infection. As such, improving our understanding of how newborns cope with moderate levels of pulmonary oedema and remove this liquid from lung tissue may improve our understanding of how to treat COVID-19 patients with severe respiratory distress.

Introduction
After birth, clearance of the liquid that resides in the airways during fetal life allows the entry of air into the lungs and the onset of pulmonary gas exchange. This in turn triggers a physiological sequence of events that underpins the transition from fetal to newborn life. While airway liquid clearance represents a major physiological challenge, the vast majority of newborn infants are able to overcome these challenges with remarkable ease. However, up to 20% of infants require some form of assistance at birth to aerate their lungs and commence air-breathing. To better help infants make this transition, we need to understand how this liquid is cleared from the lungs and how the uptake of liquid into lung tissue affects respiratory function in the newborn.
Phase contrast X-ray imaging at SPring-8 revealed how the lungs aerate at birth
While it has been known for centuries that the fetal lung is filled with liquid during pregnancy, the mechanism for its clearance from the airways at birth remained a mystery until the early 1980s. Many had postulated that this liquid was "squeezed out" of the infant during labour and vaginal delivery, which is consistent with many reported observations of liquid "gushing" from the nose and mouth upon delivery of the infant's head. However, as the head provides the greatest obstacle for delivery in humans, with the chest providing little to no resistance, this theory was largely dismissed as a mechanism for airway liquid clearance. Indeed, it doesn't explain how airway liquid is cleared in infants delivered by caesarean section as they are not exposed to labour or vaginal delivery. In the 1980s, researchers discovered that elevated adrenaline levels stimulated Na+ reabsorption from the lung lumen, which reversed the osmotic gradient across the pulmonary epithelium[1][1] R. E. Olver, D. V. Walters and S. M. Wilson: Annu. Rev. Physiol. 66 (2004) 77-101.. This led to the suggestion that the clearance of airway liquid from the lung lumen and into lung tissue during labour resulted from a stress induced activation of Na+ reabsorption. However, Na+ reabsorption-induced liquid reabsorption does not develop until late in gestation, is relatively slow and is reliant on the infant being "stressed" with elevated adrenaline levels[1][1] R. E. Olver, D. V. Walters and S. M. Wilson: Annu. Rev. Physiol. 66 (2004) 77-101.. As such, it does not explain how infants born premature (ie before this mechanism develops) or those delivered by caesarean section without the stress of labour, clear their airways of liquid at birth[2][2] S. B. Hooper, A. B. Te Pas and M. J. Kitchen: Arch. Dis. Child. Fetal Neonatal Ed. 101 (2016) F266-271.. It also does not explain how newborns can easily commence pulmonary gas exchange within 30 secs to a minute of birth, as Na+ reabsorption-induced liquid clearance rates are slow[3,4][3] S. B. Hooper, M. J. Kitchen, M. J. Wallace, N. Yagi, K. Uesugi et al.: FASEB J. 21 (2007) 3329-3337.
[4] M. L. Siew, M. J. Wallace, M. J. Kitchen, R. A. Lewis, A. Fouras et al.: J. Appl. Physiol. 106 (2009) 1888-1895.. Indeed, pharmacological doses of adrenaline can only stimulate airway liquid clearance rates at ~10 mL/h/kg and therefore it would take hours to clear the volume of liquid present in the infants' airways prior to birth (30-40 mL/kg)[2][2] S. B. Hooper, A. B. Te Pas and M. J. Kitchen: Arch. Dis. Child. Fetal Neonatal Ed. 101 (2016) F266-271..
Discovery of the primary mechanism underpinning airway liquid clearance at birth only became evident when the process was imaged using phase contrast (PC) X-ray imaging at SPring-8 (BL20B2)[3,4][3] S. B. Hooper, M. J. Kitchen, M. J. Wallace, N. Yagi, K. Uesugi et al.: FASEB J. 21 (2007) 3329-3337.
[4] M. L. Siew, M. J. Wallace, M. J. Kitchen, R. A. Lewis, A. Fouras et al.: J. Appl. Physiol. 106 (2009) 1888-1895.. PC X-ray imaging uses the refractive index difference between air and water to produce contrast that is orders of magnitude greater than the contrast produced by X-ray absorption[5][5] M. J. Kitchen, R. A. Lewis, S. B. Hooper, M. J. Wallace, K. K. W. Siu et al.: American Institute of Physics 879 (2007) 1903-1907.. As a result, the air/liquid boundaries become highly visible allowing the air-filled structures of the lung to be imaged with such high resolution, that for the first time in history, the smallest air-filled structures of the lung (alveoli) could be imaged in situ in a living breathing animal (Fig. 1). The compilation of image sequences acquired during lung aeration into videos demonstrated that pressure gradients generated during inspiration was the primary mechanism driving airway liquid clearance at birth[3,4][3] S. B. Hooper, M. J. Kitchen, M. J. Wallace, N. Yagi, K. Uesugi et al.: FASEB J. 21 (2007) 3329-3337.
[4] M. L. Siew, M. J. Wallace, M. J. Kitchen, R. A. Lewis, A. Fouras et al.: J. Appl. Physiol. 106 (2009) 1888-1895.. While being imaged, newborn rabbits were placed in a plethysmograph to record the changes in lung gas volumes simultaneously with high spatial resolution imaging, providing incontrovertible evidence for the role of inspiration[3,4][3] S. B. Hooper, M. J. Kitchen, M. J. Wallace, N. Yagi, K. Uesugi et al.: FASEB J. 21 (2007) 3329-3337.
[4] M. L. Siew, M. J. Wallace, M. J. Kitchen, R. A. Lewis, A. Fouras et al.: J. Appl. Physiol. 106 (2009) 1888-1895.. Subsequent imaging experiments at SPring-8 confirmed that the pressures generated during inspiration were responsible for > 95% of airway liquid clearance and that positive pressure inflations of the lung, which artificially replicate these pressure gradients, are equally as effective[6,7][6] M. L. Siew, A. B. Te Pas, M. J. Wallace, M. J. Kitchen, R. A. Lewis et al.: J. Appl. Physiol. 106 (2009) 1487-1493.
[7] A. B. Te Pas, M. J. Kitchen, K. Lee, M. J. Wallace, A. Fouras et al.: Pediatr. Res. 80 (2016) 85-91.. Furthermore, we demonstrated that as the liquid moves from the airways and into lung tissue, it remains within the chest and so the chest wall must expand to accommodate both the volume of incoming air as well as the liquid which residing within the tissue[3,8][3] S. B. Hooper, M. J. Kitchen, M. J. Wallace, N. Yagi, K. Uesugi et al.: FASEB J. 21 (2007) 3329-3337.
[8] E. V. McGillick, K. Lee, S. Yamaoka, A. B. Te Pas, K. J. Crossley et al.: J. Appl. Physiol. 123 (2017) 1204-1213.. This explains why infants must have a compliant chest wall at birth, which stiffens in the first few weeks after birth, to avoid compromising respiratory function in the immediate newborn period.
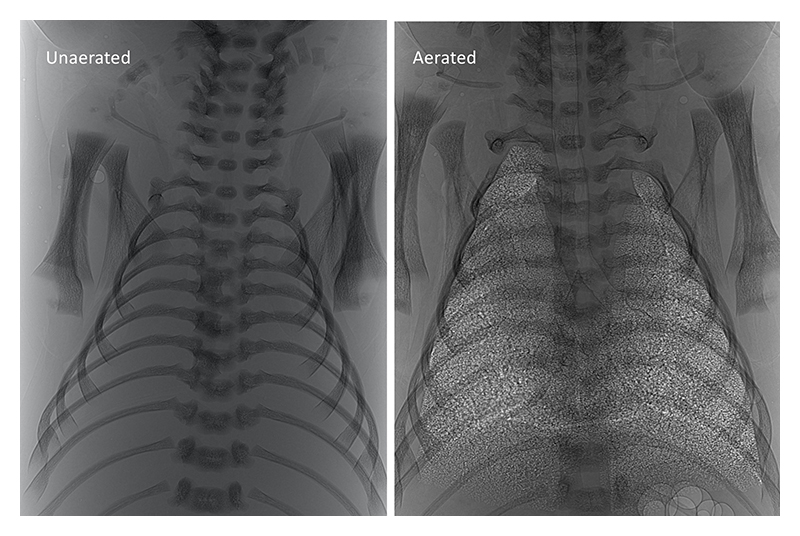
Figure 1 Phase contrast X-ray image of a newborn rabbit kitten before (left) and after (right) lung aeration.
Respiratory consequences of airway liquid clearance at birth
While respiratory distress (RD) in newborn infants is commonly associated with premature birth and immature lungs, it is more common in mature infants born at or near term, particularly in those delivered by elective caesarean section. These infants are born healthy, but gradually develop RD in the first 1 to 2 hours after birth, requiring admission into intensive care. This form of RD is characterised by tachypnea (increased breathing rate), hypoxia (low oxygen levels) and grunting during breathing. In the majority of infants, this form of RD is mild and self-resolving after a few hours and is commonly referred to as transient tachypnoea of the newborn (TTN) or "wet lung" [9][9] L. Jain and G. G. Dudell: Seminars in Perinatology 30 (2006) 296-304.. However, in severe cases, RD in mature infants can be life threatening and for > 40 years, was widely considered to be caused by an inability to clear the airways of liquid[1][1] R. E. Olver, D. V. Walters and S. M. Wilson: Annu. Rev. Physiol. 66 (2004) 77-101.. An inability to clear airway liquid in the absence of labour was thought to explain the 4-fold higher prevalence of RD observed in infants delivered by elective caesarean section who do not experience the "stress" of labour[9][9] L. Jain and G. G. Dudell: Seminars in Perinatology 30 (2006) 296-304.. As such, adrenaline-induced activation of Na+ reabsorption would be reduced or absent, which would theoretically impede airway liquid clearance. However, our discovery made at SPring-8 (BL20B2) using PC X-ray imaging, demonstrated that the pressures generated by inspiration are primarily responsible for airway liquid clearance. Thus, as term infants with RD have increased breathing rates, they have an increased capacity to clear airway liquid.
In another discovery at SPring-8 (BL20B2) using PC X-ray imaging, we showed that the presence of larger volumes of liquid in the airways adversely impacts on respiratory function in the newborn and that this adverse effect was greater in more mature newborns delivered near term[7][7] A. B. Te Pas, M. J. Kitchen, K. Lee, M. J. Wallace, A. Fouras et al.: Pediatr. Res. 80 (2016) 85-91. (proposal No. 2018A0150-2019B0150). This discovery uncovered a new mechanism to explain the RD in near term infants (term RD)[8][8] E. V. McGillick, K. Lee, S. Yamaoka, A. B. Te Pas, K. J. Crossley et al.: J. Appl. Physiol. 123 (2017) 1204-1213.. That is, the presence of large volumes of airway liquid at the onset of air-breathing at birth requires all of that liquid to be cleared from the airways into lung tissue, causing pulmonary oedema. Logically, as the volume of airway liquid at birth increases, the degree of pulmonary oedema will worsen.
Consequences of elevated airway liquid volumes at birth
To investigate the theory that elevated airway liquid volumes at birth are largely responsible for term RD, we conducted further PC X-ray imaging studies at SPring-8. These studies have allowed us to define the full respiratory consequences of elevated airway liquid volumes at birth, providing compelling physiological evidence that greater airway liquid volumes at birth induce term RD. Specifically, we have shown that increased airway liquid volumes at birth:
1. Increase chest wall expansion and flatten the diaphragm, which reduces the newborn's ability to inspire and increases the work of breathing (Fig. 2A&B).
2. Reduces end-expiratory lung gas volumes between breaths (functional residual capacity, FRC), which is a localised effect specifically caused by the elevated liquid. Fig. 3 shows the effect of elevated liquid in the right lung only on FRC (Fig. 2C).
3. Reduce lung compliance, which contributes to reduced FRC and also limits inspiration (Fig. 2D).
4. Increases the need for supplemental oxygen to maintain oxygenation due to reduced gas exchange capacity (Fig. 2E).
5. Induces tachypnea (increased breathing rate) and expiratory braking, which is used to defend end-expiratory lung volumes, in spontaneously breathing newborns.
These findings have allowed us to develop the first ever animal model that recapitulates all of the respiratory morbidity characterised by term RD in spontaneously breathing newborn rabbits and sheep. Furthermore, it allowed us to propose strategies that may reduce the risk and/or severity of term RD in infants delivered by caesarean section.

Figure 2 Elevated liquid volumes at the onset of air-breathing; (A) increases chest expansion as indicated by an increase in chest wall area, (B) flattens the diaphragm as indicated by an increase in the radius of curvature, (C) reduces the volume of gas retained within the lung at end-expiration, (D) greatly reduces lung compliance, and (E) increases the inspired oxygen content that is required to maintain oxygenation levels in the newborn.

Figure 3 A comparison of aeration in the left (L) and right (R) lung, with the left having a normal level of lung liquid and the right having an elevated level of lung liquid at ventilation onset. This shows that the effect of elevated liquid on lung is regionally specific.
Implications of our findings and further studies
Our PC X-ray imaging studies at SPring-8 has prompted a new understanding of the causes and consequences of airway liquid clearance at birth. Our results clearly demonstrate that following birth, airway liquid is cleared from the airways and into lung tissue due to the pressure gradients generated during breathing. As such, all liquid present in the airways at birth must move from the airways into lung tissue. As this causes pulmonary oedema, it has the potential to adversely impact respiratory function, despite a highly compliant and expandable chest wall. Thus, term RD likely arises when the volume of airway liquid exceeds the volume of liquid that can be moved into lung tissue without increasing the level of oedema to the point that it adversely impacts on lung function.
Irrespective of whether airway liquid is cleared via Na+ reabsorption or via pressure gradients generated by inspiration, liquid cleared via these mechanisms must enter lung tissue, which risks the development of pulmonary oedema[2][2] S. B. Hooper, A. B. Te Pas and M. J. Kitchen: Arch. Dis. Child. Fetal Neonatal Ed. 101 (2016) F266-271.. The only other mechanism is liquid clearance via the nose and mouth during labour and vaginal delivery and, as a result, liquid does not enter lung tissue. While this mechanism is unlikely to result from "squeezing" the chest during delivery as previously suggested, an increase in dorso-ventral (knees to chest) flexion of the infant's trunk could account for increased liquid loss from the airways during uterine contractions as previously described[2][2] S. B. Hooper, A. B. Te Pas and M. J. Kitchen: Arch. Dis. Child. Fetal Neonatal Ed. 101 (2016) F266-271.. While this mechanism cannot account for all the liquid cleared from the airways, the contribution of this mechanism ensures that the volume of liquid that must be cleared into lung tissue after birth is substantially reduced. As a result, we are now undertaking a clinical trial to determine whether a knees-to-chest flexion of infants during caesarean section delivery reduces the risk of TTN after birth in infants delivered via this approach.
Our PC X-ray imaging studies at SPring-8 have provided definitive evidence that pulmonary oedema is the primary underlying cause of the RD suffered by mature infants born at or near term[7,8][7] A. B. Te Pas, M. J. Kitchen, K. Lee, M. J. Wallace, A. Fouras et al.: Pediatr. Res. 80 (2016) 85-91.
[8] E. V. McGillick, K. Lee, S. Yamaoka, A. B. Te Pas, K. J. Crossley et al.: J. Appl. Physiol. 123 (2017) 1204-1213.. It is also the underlying cause of the severe RD suffered by patients infected with the COVID-19 virus. However, we know very little about the pathophysiology of severe pulmonary oedema in this context and how the lung clears this liquid from lung tissue. At rest, lung interstitial tissue pressures are normally sub-atmospheric, which plays an important role in stabilising small airways, capillaries and lymphatic vessels. These structures have internal luminal pressures that are atmospheric or slightly above atmospheric, with the capillaries and lymphatics being the primary suspected route for liquid clearance from lung tissue. As the patency of these delicate structures are assisted by the sub-atmospheric pressure in the surrounding lung tissue, when interstitial tissue pressures increase due to oedema, these vessels collapse and the alveoli can re-flood. As a result, the only known routes for clearing this liquid from lung tissue close, trapping the liquid within the lung.
Very little is known about liquid clearance from lung tissue after birth and the factors that regulate this process but is clearly a critical piece of missing information. In recent experiments at SPring-8, we have developed a 3D computed tomography (CT) technique for tracking airway liquid as it leaves the airways and enters lung tissue after birth, providing the first definitive proof for liquid movement into lung tissue (Fig. 4). Using this approach, we plan to investigate the pathways and the factors that influence liquid movement from lung tissue and how this is influenced by the level of pulmonary oedema.
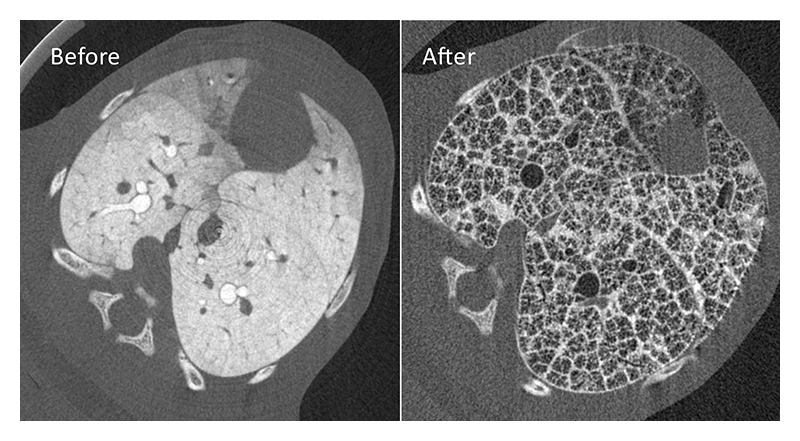
Figure 4 Computed tomography (CT) slices of the same lung in a rabbit kitten before and after lung aeration. An X-ray contrast agent was added to lung liquid before lung aeration to track liquid movement out of the airways and into lung tissue and then it's clearance from lung tissue.
Conclusion
While the problems associated with airway liquid clearance at birth were considered to be uniquely relevant to newborn infants, it is becoming increasingly apparent that the underlying pathophysiology may also be relevant to COVID-19 patients with severe RD. The studies we have undertaken at SPring-8 have led to the discovery of how airway liquid is primarily cleared at birth and to a new understanding for the RD distress suffered by normal healthy infants born at or near term. We have collected compelling evidence to indicate that elevated airway liquid levels at the onset of air-breathing at birth leads to a level of pulmonary oedema that compromises respiratory function after birth. However, understanding how the lung clears this liquid from lung tissue will allow the development of treatments that not only benefit newborn infants but may also greatly increase survival in severely affected COVID-19 patients.
References
[1] R. E. Olver, D. V. Walters and S. M. Wilson: Annu. Rev. Physiol. 66 (2004) 77-101.
[2] S. B. Hooper, A. B. Te Pas and M. J. Kitchen: Arch. Dis. Child. Fetal Neonatal Ed. 101 (2016) F266-271.
[3] S. B. Hooper, M. J. Kitchen, M. J. Wallace, N. Yagi, K. Uesugi et al.: FASEB J. 21 (2007) 3329-3337.
[4] M. L. Siew, M. J. Wallace, M. J. Kitchen, R. A. Lewis, A. Fouras et al.: J. Appl. Physiol. 106 (2009) 1888-1895.
[5] M. J. Kitchen, R. A. Lewis, S. B. Hooper, M. J. Wallace, K. K. W. Siu et al.: American Institute of Physics 879 (2007) 1903-1907.
[6] M. L. Siew, A. B. Te Pas, M. J. Wallace, M. J. Kitchen, R. A. Lewis et al.: J. Appl. Physiol. 106 (2009) 1487-1493.
[7] A. B. Te Pas, M. J. Kitchen, K. Lee, M. J. Wallace, A. Fouras et al.: Pediatr. Res. 80 (2016) 85-91.
[8] E. V. McGillick, K. Lee, S. Yamaoka, A. B. Te Pas, K. J. Crossley et al.: J. Appl. Physiol. 123 (2017) 1204-1213.
[9] L. Jain and G. G. Dudell: Seminars in Perinatology 30 (2006) 296-304.